Thermodynamics- Gibbs free energy
In thermodynamics, Gibbs free energy (often referred to simply as Gibbs energy, symbolized as G) is a thermodynamic potential that quantifies the maximum non-pressure-volume work achievable by a thermodynamically isolated system at constant temperature and pressure. It also serves as a criterion for processes, including chemical reactions, that can occur under these conditions.
What is Gibbs free energy?
As per the second law of thermodynamics, when systems react at a constant temperature and pressure without the addition of non-pressure-volume (pV) work, they tend to naturally minimize their Gibbs free energy.
The Gibbs free energy, denoted as G(p,T), is a measure used in thermodynamics to determine the maximum reversible work that may be performed by a system at a constant temperature and pressure. It’s defined by the formula:
G(p,T) = U + pV – TS
Where:
- U is the internal energy of the system (measured in joules).
- p is the pressure of the system (measured in pascals).
- V is the volume of the system (measured in cubic meters).
- T is the temperature of the system (measured in kelvin).
- S is the entropy of the system (measured in joules per kelvin).
The change in Gibbs free energy (Δ G ) is related to the change in enthalpy (ΔH ) and the change in entropy (ΔS) through the equation:
ΔG = Δ H – T ΔS
Additionally, the heat transferred (ΔQ ) at constant temperature is given by:
ΔQ = T ΔS
What is the significance of Gibbs free energy?
In simple terms, Gibbs free energy tells us whether a reaction will occur spontaneously. If ΔG < 0, the reaction is spontaneous and can release energy. If ΔG > 0, the reaction is non-spontaneous and requires input of energy to occur. If Δ= 0, the system is at equilibrium. Gibbs free energy is a crucial thermodynamic function for system characterization, influencing outcomes such as the voltage of an electrochemical cell and the equilibrium constant of a reversible reaction.
What does a positive delta G mean?
The ΔG for a reaction represents the change in Gibbs free energy. A negative ΔG indicates that the reaction is spontaneous which is referred to as exergonic. A positive ΔG indicates that the reaction is non-spontaneous.
Spontaneous processes
In chemistry, a spontaneous process is defined as one that proceeds without the need for external energy input. The speed at which a spontaneous process occurs can vary, as spontaneity is independent of reaction kinetics or rate. An illustrative example is the transformation of carbon from diamond to graphite, represented by the following reaction:
C(s,diamond) -> C(s,graphite)
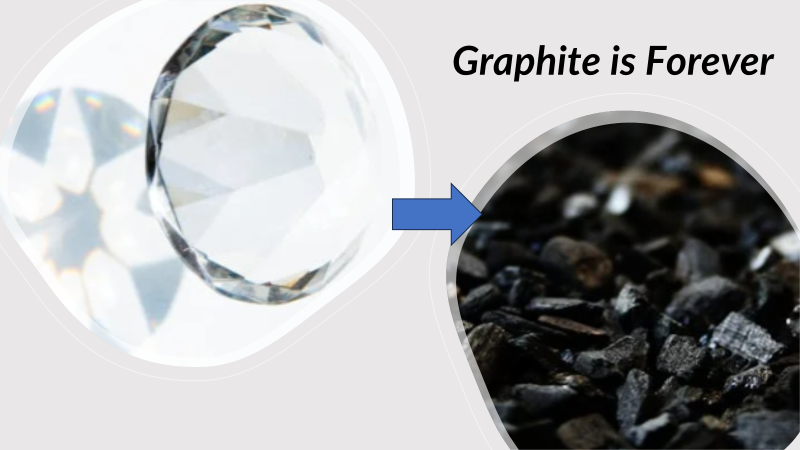
What is Enthalpy?
The sum of the internal energy and the pressure-volume product of a thermodynamic system. Imagine you have a system, like a pot of water boiling on the stove. Enthalpy is like the total energy of the water, including not just its heat but also the energy needed to make space for it in the air around the pot. This extra energy accounts for the work required to push the air out of the way as the water heats up and expands, especially if the pressure stays the same.
What is Entropy
Think of entropy as a measure of how messy or disorganized things are within a system. When a system is highly organized, its entropy is low. But as things become more random or disordered, the entropy increases.
Another way to look at entropy is as a measure of the energy in a system that can’t be used to do work. This energy is kind of “locked away” in the disorder of the system and can’t be harnessed efficiently. So, in a sense, entropy tells us how much of a system’s energy is essentially wasted in terms of doing useful work.
What is Hess’s Law
Hess’s Law is a fundamental principle in thermodynamics that states the enthalpy or heat change in a chemical reaction is independent of the pathway taken from the initial to the final state. In simpler terms, it means that if a chemical change occurs through several different routes, the overall enthalpy change remains the same, as long as the initial and final conditions are the same.
This principle allows chemists to calculate the enthalpy change (ΔH ) for a reaction even when it cannot be directly measured. This is done by using basic algebraic operations based on the chemical equation of reactions and utilizing previously determined values for the enthalpies of formation. Hess’s Law is a powerful tool in understanding and predicting the energy changes in chemical reactions, providing a consistent framework for studying the thermodynamics of chemical systems.
Example 1
- Cgraphite+O2 → CO2 (g) ; (ΔH = -393.5 kJ) (direct step)
- Cgraphite+1/2 O2 → CO (g) ; (ΔH = -110.5 kJ)
- CO (g)+1/2 O2 → CO2 (g); (ΔH = – 283.02 kJ)
In reactions 2 and 3, the total ΔH = -393.5 kJ which is equal to ΔH in 1. The difference in the value of ΔH is 0.02 kJ which is due to measurement errors.
Example 2
Find Gibbs free energy for the following system:
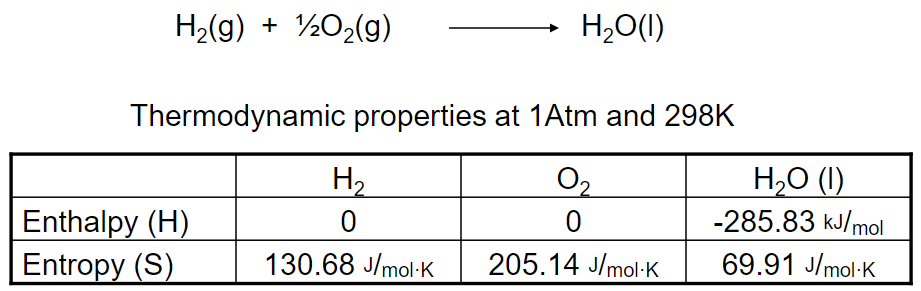
Solution
Enthalpy of the chemical reaction using Hess’ Law:
ΔH = ΔHreaction = ΣHproducts – ΣHreactants
= (1mol)(-285.83 kJ/mol) – (0)
= -285.83 kJ
The entropy of chemical reaction:
ΔS = ΔSreaction = ΣSproducts – ΣSreactants
= [(1mol)(69.91 J/mol·K)] – [(1mol)(130.68 J/mol·K) + (½mol)(205.14 J/mol·K)]
= -163.34 J/K
Heat gained by the system:
ΔQ = TΔS
= (298K)(-163.34 J/K)
= -48.7 kJ
The Gibbs free energy is then calculated by:
ΔG = ΔH – TΔS
= (-285.83 kJ) – (-48.7 kJ)
= -237 kJ
Answer
The work done on the reaction by the environment is: W = ΔG = -237 kJ
The heat transferred to the reaction by the environment is: ΔQ = TΔS = -48.7 kJ
More simply stated: The chemical reaction can do 237 kJ of work and produce 48.7 kJ of heat to the environment.
Gibbs Free Energy and Fuel Cells
The Gibbs Free Energy (G) is a key concept in thermodynamics, often used to predict the spontaneity of chemical reactions. However, its applications extend beyond this fundamental role. One notable application is in the calculation of the output voltage of an ideal fuel cell.
In a fuel cell, chemical energy is converted directly into electrical energy through a redox reaction. The Gibbs Free Energy change (ΔG ) for this reaction is related to the cell voltage (E) through the equation:
ΔG=−nFE
Where:
- ( n ) is the number of electrons transferred per reaction.
- ( F ) is the Faraday constant (approximately 96,485 C/mol).
- ( E ) is the cell voltage.
The cell voltage (E) can be calculated as the difference between the standard half-cell potentials (E°) of the two half-reactions involved in the fuel cell. This is a key concept in understanding the efficiency and performance of fuel cells, as the voltage directly relates to the energy output of the cell.
By using Gibbs Free Energy in conjunction with the Faraday constant and the number of electrons transferred in the reaction, scientists and engineers can design and optimize fuel cell systems for various applications, ranging from portable electronics to large-scale power generation. This demonstrates the versatility and importance of thermodynamic principles, such as Gibbs Free Energy, in understanding and advancing energy conversion technologies.
Theoretical specific energy
Theoretical specific energy, measured in J/g or Wh/kg, quantifies the energy stored per unit weight in a battery or fuel cell. It is calculated as the product of the theoretical cell voltage and the specific charge.
Example: NiFe
Basis for calculation: 1 mole
Free energy change (ΔG) during discharge
G = -nFE = -2 * 96500 * 1.26 = -243180 J = -243.2 kJ
=> -67.55 Wh/mol
The total mass of reactants
Fe(OH)2 + … Ni(OH)2 <=> (1/3) Ni3O4 + … Fe
Fe(OH)2 = 89.9 g
Ni (OH)2 = 92.7 g
Total mass 182.6 g => 0.183 kg/mol
Theoretical specific energy
67.6 Wh/ 0.183 kg = 369.9 Wh/kg
Example: Pb-acid
Basis for calculation: 1 mole
Free energy change (ΔG) during discharge
G = -nFE = -2 * 96500 * 2.05 = -395700 J = -395.7 kJ => -109.9 Wh/mol
The total mass of reactants
PbO2 + Pb + 2H2SO4 <=> 2PbSO4 + 2H20
PbO2 = 239 g
Pb = 207 g
2H2SO4 = 98 g *2 = 196 g
Total mass 642 g => 0.642 kg/mol
Theoretical specific energy
109.9 Wh/ 0.642 kg = 171 Wh/kg
Example: LiCoO2 – C
Basis for calculation: 1 mole
Free energy change (ΔG) during discharge
G = -nFE = -2 * 96500 * 4.0 = -386000 J = -386.0 kJ => -107.2 Wh/mol
The total mass of reactants
CoO2 + LiC6 <=> LiCoO2 + C6
CoO2 = 91 g
LiC6 = 79 g
Total mass 170 g => 0.170 kg/mol
Theoretical specific energy
107.2 Wh/ 0.170 kg = 630.6 Wh/kg
Conclusion
In conclusion, Gibbs free energy is a fundamental concept in thermodynamics, offering valuable insights into the spontaneity and feasibility of chemical reactions. Its application extends beyond chemistry, playing a crucial role in various fields, including biology and environmental science.
By understanding Gibbs free energy, scientists and engineers can make informed decisions in designing energy-efficient processes and developing sustainable technologies. As we continue to explore the complexities of thermodynamics, the significance of Gibbs free energy remains paramount in our quest for a deeper understanding of the physical world.