Solar Cell Basics
A solar cell is a device that converts light energy into electrical energy using the photovoltaic effect. Most solar cells are made from silicon. Unlike batteries or fuel cells, solar cells do not rely on chemical reactions or fuel, and unlike electric generators, they have no moving parts. Solar cells can be organized into solar panels, which consist of numerous individual solar cells. These arrays can serve as centralized electric power stations, converting sunlight into electricity for industrial, commercial, and residential use.
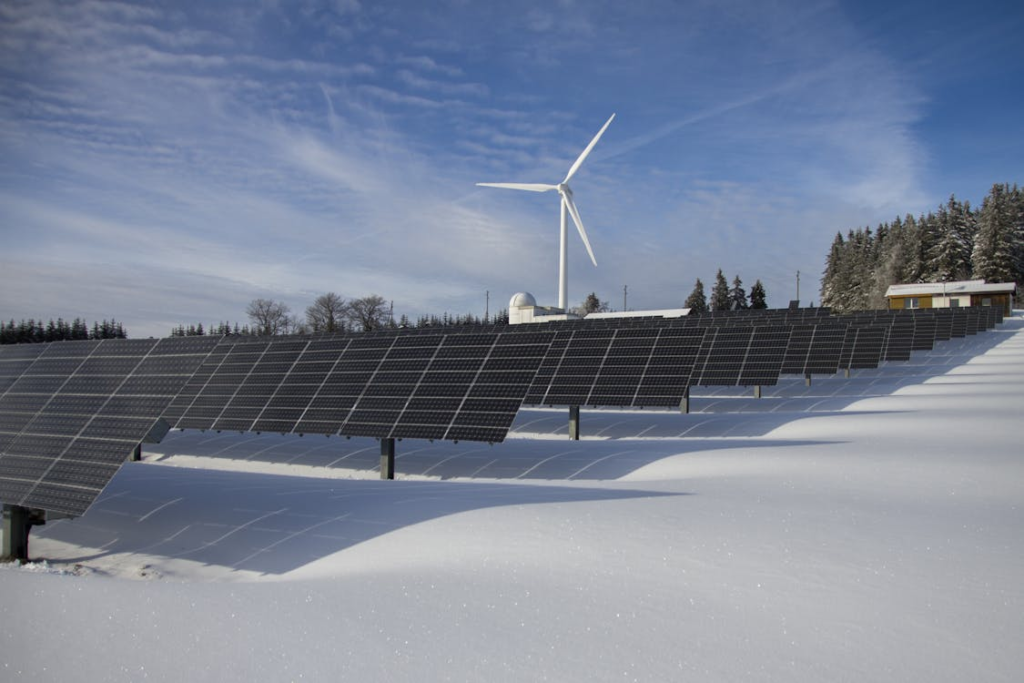
What is a solar cell?
A solar cell is a photovoltaic device that converts solar energy into electrical energy based on the principles of the photovoltaic effect. It is part of photovoltaic (PV) systems that directly convert light energy into electricity. Simple PV systems power small calculators, while more complex systems can provide a significant amount of electricity. Solar cells are a promising means of maintaining our energy-intensive standard of living without contributing to global warming and pollution.

What is the average life of a solar cell?
The majority of PV systems are relatively new, with around 70% of solar energy systems installed since 2017. While the estimated operational lifespan of a PV module is about 30-35 years, some may continue to function for much longer.
What is the difference between solar panels and solar cells?
Solar cells form the foundation of solar panels, with several cells oriented uniformly to create a panel. These cells generate electricity through the photovoltaic effect, a natural process.
Solar Cells History
The history of the solar cell dates back to 1839 when French physicist A. E. Becquerel first recognized the photovoltaic effect, which is the ability of certain materials to convert light into electricity. This discovery laid the foundation for the development of solar cells. The term “photovoltaic” is derived from the words “photo,” meaning light, and “voltaic,” referring to electricity.
In 1883, Charles Fritts constructed the first solar cell by coating a semiconductor with an extremely thin layer of gold. This formed the junctions necessary for the photovoltaic effect. Although inefficient by modern standards, this early solar cell was a significant milestone in the development of photovoltaic technology.
The real breakthrough in solar cell technology came in 1954 when researchers at Bell Laboratories discovered that silicon doped with certain impurities was highly sensitive to light. Daryl Chapin, Calvin Fuller, and Gerald Pearson invented the first practical device for converting sunlight into electrical power. This led to the production of the first practical solar cells with a sunlight energy conversion efficiency of around 6%. The history of the solar cell is a testament to human ingenuity and the pursuit of clean and renewable energy.
Materials for Solar cell
Solar cells are built using a variety of semiconducting materials, each with its unique properties and advantages. One of the most common materials used is crystalline silicon, which has been used in solar cells for many years. Another popular material is cadmium telluride, known for its efficiency and low manufacturing costs.
Copper indium diselenide is also used, offering good efficiency and flexibility. Gallium arsenide is highly efficient but more expensive, making it suitable for specialized applications. Indium phosphide and zinc sulfide are used in more advanced solar cell technologies. These materials offer high efficiency but at a higher cost. These materials are also chosen for their ability to become electrically conductive when exposed to light. Thus allowing them to convert solar energy into electricity efficiently.
PV Solar for Electricity
Photovoltaic (PV) solar technology offers a promising solution for providing electricity to regions without access to a traditional power grid. For the approximately 2 billion people worldwide lacking access to electricity, installing solar panels can be more cost-effective than extending the electrical grid. This approach is supported by organizations like The Fund for Renewable Energy Everywhere.
A significant and fast-growing market for PV solar is in providing power to villages in developing countries. The United Nations estimates that over 2 million villages globally lack electricity for basic needs. Solar technology could help them with pumping water, refrigeration, and lighting. The cost of extending utility grids to these villages is prohibitively high, ranging from $23,000 to $46,000 per kilometer.
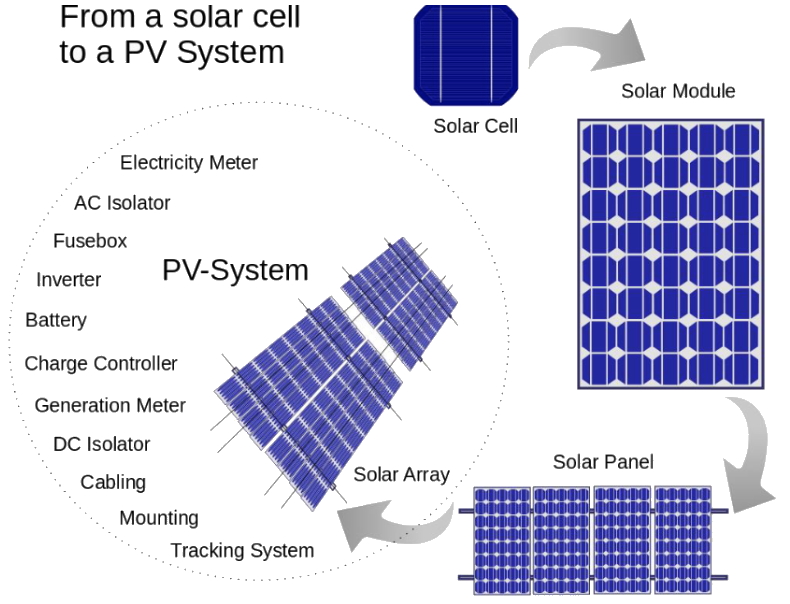
In addition to providing access to electricity, PV solar systems offer environmental benefits. For example, a one-kilowatt PV system in Colorado, or an equivalent system that produces 150 kWh per month, can prevent the mining of 150 pounds of coal, the release of 300 pounds of CO2 into the atmosphere, and the emission of NO and SO2 into the environment. These environmental benefits make PV solar a sustainable and eco-friendly energy solution for both remote communities and urban areas.
Basic Physics of Solar Cells
A solar cell operates on the principles of a p-n junction, similar to a diode but with a larger area. When silicon (Si) doped with p-type impurities is placed next to a region of Si-doped with n-type impurities, a p-n junction is formed. In this junction, holes from the p-type side diffuse to the n-type side, and electrons diffuse to the p-type side due to the concentration gradient.
This movement of charge carriers creates an electric field across the junction, making it easy for current to flow from the n-type side to the p-type side (forward bias), but difficult in the reverse direction (reverse bias). When sunlight hits the solar cell, it generates electron-hole pairs in the region near the junction. The electric field in the p-n junction separates these electron-hole pairs, pushing the electrons toward the n-type side and the holes toward the p-type side.
By connecting an external circuit to the solar cell, the separated charge carriers can flow through the circuit, generating electric power. This process allows the solar cell to convert sunlight directly into electrical energy.
How does an electron-hole pair form?
Electron-hole pairs form when photons of light strike a semiconductor material, such as the material used in a solar cell. Photovoltaic energy conversion relies on the number of photons that strike the Earth. A photon is a flux of light particles. On a clear day, approximately 4.4 x 10^17 photons strike a square centimeter of the Earth’s surface every second.
However, not all of these photons can be converted into electricity by the solar cell. Only photons with energy greater than the band gap of the semiconductor material can be converted. When a photon with sufficient energy enters the semiconductor material, it may be absorbed by an electron in the valence band, causing the electron to jump to the conduction band. This process creates an electron-hole pair, where the electron is in the conduction band and the hole (the absence of an electron) is in the valence band. These electron-hole pairs are then separated by the electric field in the solar cell, generating an electric current that can be used as electricity.
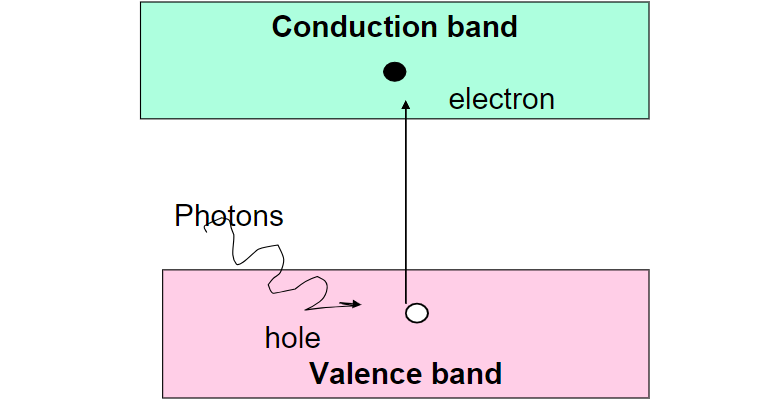
When a photon with enough energy is absorbed by a semiconductor, it can excite an electron from the valence band to the conduction band, leaving behind a vacancy in the valence band. This vacancy is known as a hole. The electron in the conduction band and the hole in the valence band can then combine, effectively recombining and neutralizing each other, forming an electron-hole pair. This recombination process releases energy, often in the form of heat, and is typically undesirable in solar cells as it reduces the efficiency of the device.
p-n Junctions
When two differently doped semiconductor layers are combined, a p-n junction is formed at the boundary between the layers. This junction is a key component of many semiconductor devices, including diodes and solar cells.
To create a p-type semiconductor, a trivalent element is used as a dopant. These elements have three valence electrons, which can create “holes” in the crystal lattice where an electron is missing. These holes act as positive charge carriers in the material.
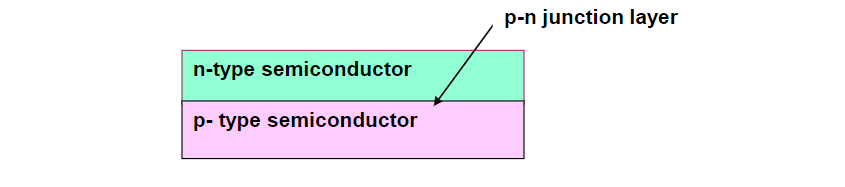
On the other hand, an n-type semiconductor is created by doping with a pentavalent element, which has five valence electrons. This introduces extra electrons into the crystal lattice, which can move freely and act as negative charge carriers.
At the interface of the p-type and n-type regions, a depletion region forms where there are no free charge carriers. This depletion region creates a built-in electric field, which plays a crucial role in the operation of semiconductor devices.
Photovoltaic effect
The photovoltaic effect is the phenomenon where a voltage is generated across a semiconductor’s p-n junction when it absorbs light radiation. This effect is the basis for photovoltaic devices, which convert light energy into electrical energy. When light photons strike the semiconductor material, they transfer their energy to electrons, allowing them to move across the p-n junction and create a voltage difference. This voltage can then be used to power electrical devices or stored for later use. Photovoltaic devices, such as solar panels, harness this effect to produce electricity from sunlight.

Construction of a solar cell
A solar cell is constructed with a sandwiched arrangement of an n-type semiconductor layer and a p-type semiconductor layer, creating a p-n junction where the two layers meet. This junction is crucial for the functioning of the solar cell, as it allows for the separation of electron-hole pairs generated by the absorption of light. To enhance the efficiency of light absorption, the surface of the cell is often coated with an anti-reflection coating, which helps to minimize the loss of incident light energy due to reflection. Additionally, proper metal contacts are made on the n-type and p-type sides of the semiconductor to provide electrical connections for the generated electricity to be collected and used externally.
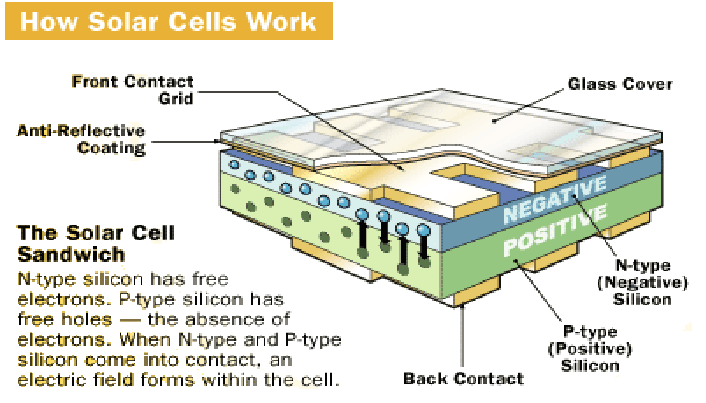
Conclusion
In conclusion, understanding the basics of solar cells is essential in grasping the remarkable technology behind solar energy. From the photovoltaic effect to the construction of solar panels, these fundamental principles illuminate how sunlight can be harnessed to generate clean and sustainable electricity. As the world increasingly looks towards renewable energy sources, solar cells stand out as a key player in shaping a greener and more sustainable future.