The shocking truth about electric forces and fields.
Introduction
Hey guys! Today, we’re going to delve into the incredible world of electric and magnetic forces. Buckle up, because we’re about to uncover some shocking truths that will blow your mind. From electric charges to magnetic fields, these forces shape the very fabric of our reality. So, let’s jump right in!
What is an electric charge simple definition?
Objects may have a property called electric charge (usually just called charge). The SI unit of
electric charge is 1 coulomb = 1 C. An electric charge is a fundamental property of matter that can be positive or negative. It is responsible for the interaction between objects through electric forces, where like charges repel and opposite charges attract.
What is conservation of charge for dummies?
The principle of conservation of charge is that the total charge in an isolated system does not change. In other words electric charge is neither created nor destroyed; it is simply transferred or redistributed “what goes in, must come out” rule for electric charges.
What is charge quantization in simple words?
Charge quantization is the concept that electric charge exists only in discrete, indivisible units. It means that charge cannot be divided into smaller fractions but occurs in multiples of a fundamental charge unit. In other words the quantization of charge is that the total charge of anything has only certain allowed
values.
What is electric current in simple words?
Electric current is the motion of electric charge through a conductor, such as a wire. The SI unit of electric current is 1 ampere = 1 amp = 1 A: 1 C ≡ 1 A·s (≡ is the mathematical symbol for “is defined to equal”).
There are two kinds of charge, positive (+) and negative (–).

Neutral objects have zero total charge, which may result from equal magnitudes of positive and negative
charges. We charge an object by adding or removing electrically-charged particles (usually electrons). Charges of the same sign repel one another. Charges of opposite sign attract one another
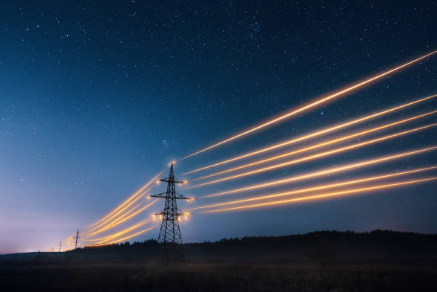
What determines whether a material is a conductor semiconductor or insulator?
The physical properties of a material determines its electrical conductivity and will determine if a engineering material is either a conductor, semiconductor, or insulator. Conductors have a high concentration of free electrons, As a result conductors have many charge carriers free to carry current. For example, copper and aluminum have about 1029 free electrons/m3.
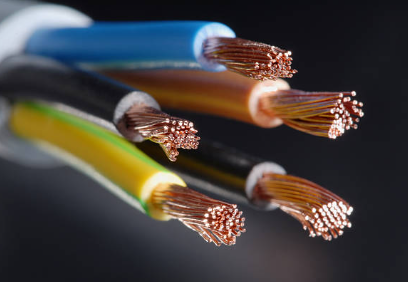
A semiconductors have a moderate concentration, as a result semiconductor has some free charge carriers. Examples include silicon, germanium, and gallium arsenide.
While insulators have minimal free electrons, affecting their conductivity properties. An insulator has hardly any free charge carriers. Examples include glass and rubber.
What is the Coulomb’s law?
Coulomb’s law states that the force between two electric charges is directly proportional to the product of their magnitudes and inversely proportional to the square of the distance between them. (The equation below is for two point charges in vacuum ≈ air)
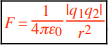
F is the magnitude of the electric force (in N = newton) on either point charge. Recall that the
magnitudes of vectors are never negative. (You find the direction of the force F→ from “Charges of the same sign repel one another. Charges of opposite sign attract one another.) ε0 is a constant:

q1 and q2 are the charge values of the two point charges (in C = coulomb). r is the separation (in m = meter) of the two point charges (r is never negative). Recall that the total force on a body is the vector sum of the individual forces on it
A charged object attracts a neutral one because, since the opposite-sign attracting charges in the two objects are closer together and the same-sign repelling charges are farther apart, there is more attraction than repulsion. For the same reason, the neutral object attracts the charged one with a force that is equal in magnitude but opposite in direction.
How does an object of charge q attract or repel an object of charge q0?
- Step 1: The first object, because it has charge q, sets up an electric field E in the space around that object.
- Step 2: The second object, because it has charge q0, experiences a force F0 from that electric field .
What is an electric field in simple terms?
An electric field is a region around an electric charge or collection of charges where a force can be exerted on other charged objects. It describes the influence or effect that the charge has on its surroundings, causing electrically charged particles to experience a force when placed in the field.
We define the electric field (E) at any point to be the electric force per charge F0/q0 on a test charge q0 placed at that point: E is in N/C Thus vector equations are

and

where E is an external electric field. (That is, E is not the electric field of q0 itself.) These vector equations tell us that E and F0 are in the same direction if q0 is positive (+), but E and F0 are in opposite directions if q0 is negative (–). To find the magnitude E of the electric field of a point charge of absolute charge value |q|.

If the charge q is positive (+), the electric field E it sets up is away from it, but if the charge q is negative (–), the electric field E it sets up is toward it. The total electric field at any point is the vector sum of the individual electric fields at that point.
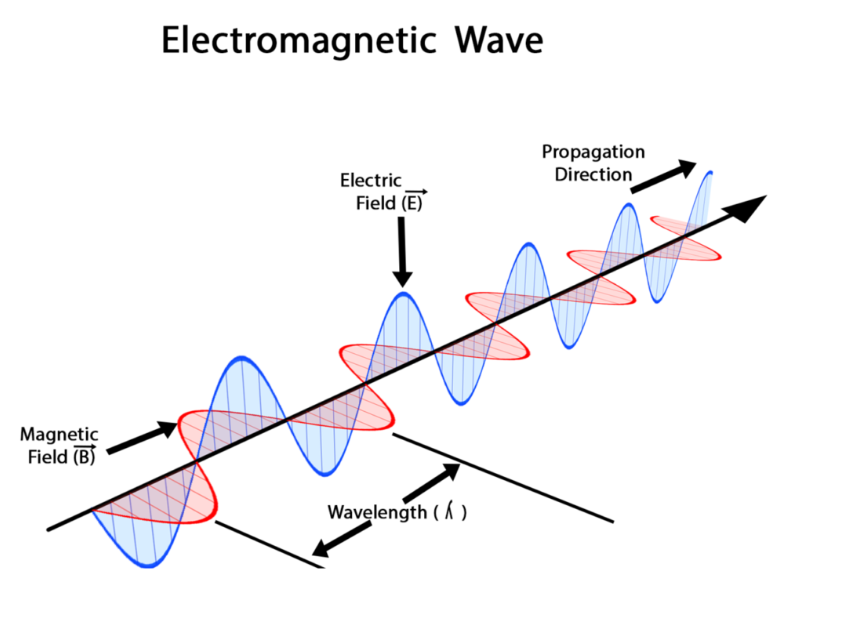
For a continuous distribution of charge:

Electric field lines:
- Used to visualize the electric field. (See many figures in this and following chapters.)
- E is tangent to an electric field line at any point.
- E is larger where those lines are closer together (and smaller where they are farther apart).
- An electric field line can be said to start on a positive charge and end on a negative charge.
Conclusion
In conclusion, the world of electric and magnetic forces is truly astonishing and holds many shocking truths. From the fascinating interactions of electric charges to the invisible power of magnets, these forces shape our daily lives in ways we might not even realize. The conservation of charge, the behavior of conductors, semiconductors, and insulators, and the quantization of charge all contribute to our understanding of how these forces operate. Moreover, Coulomb’s law provides us with a mathematical foundation to comprehend the strength of these forces in various scenarios.
Electricity and magnetism are not just concepts confined to physics textbooks; they are an integral part of the technological marvels that surround us. From electrical appliances to advanced medical devices, our world owes a tremendous debt to these forces. Understanding electromagnetism has revolutionized industries and communication, bringing about an era of technological advancement.