Hybrid Electric Vehicles
Hybrid electric vehicles (HEVs) are driven by both an internal combustion engine and one or more electric motors, utilizing energy stored in batteries. Unlike fully electric vehicles and Plug in Hybrids (PHEVs), HEVs cannot be directly plugged in to charge their batteries. Instead, the battery is recharged through regenerative braking and by the internal combustion engine.
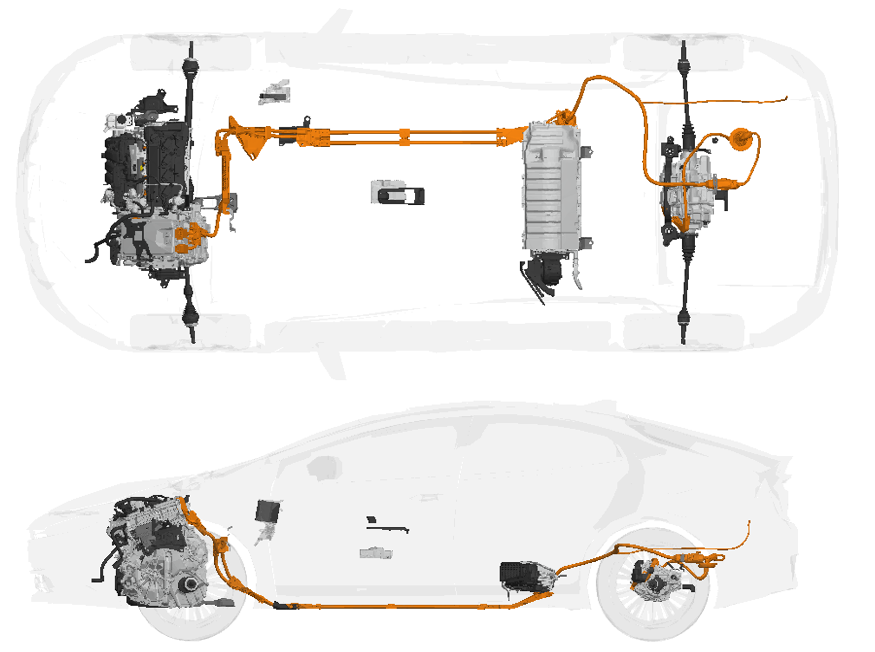
The additional power from the electric motor can enable the use of a smaller engine. Furthermore, the battery can supply power to auxiliary loads and reduce engine idling when the vehicle is stopped. These combined features lead to improved fuel efficiency without compromising performance.
History of Hybrid Electric Vehicles
Hybrids entered the U.S. market in the 2000 model year with the introduction of the Honda Insight, followed closely by the Toyota Prius, which had been available in Japan since 1997. Initially, โhybridโ referred to small, fuel-efficient sedans.
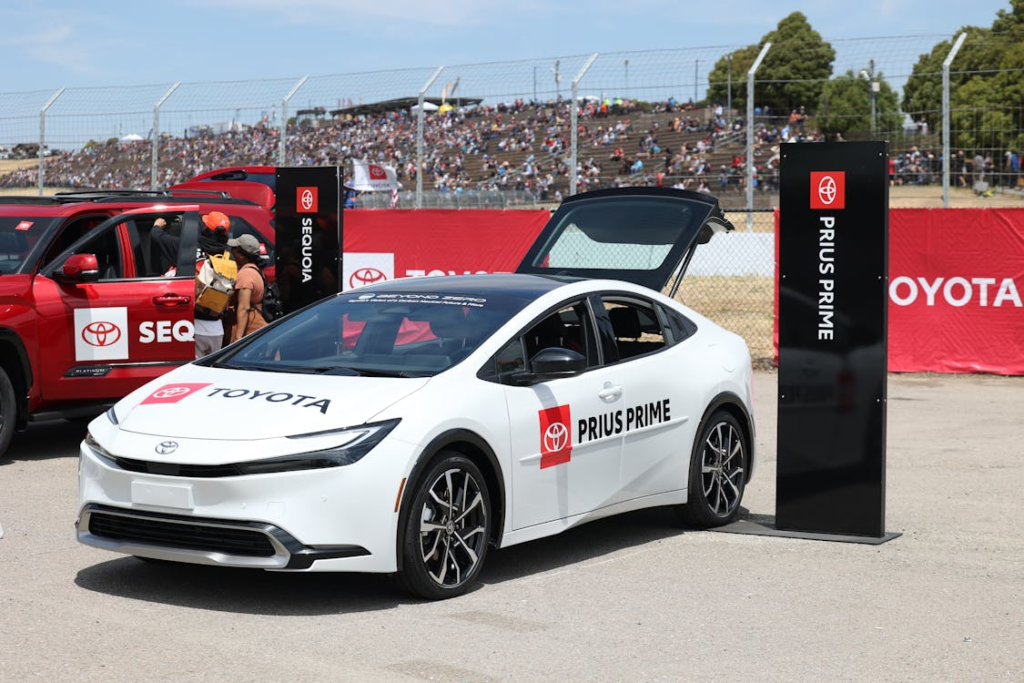
Since then, hybrid technology has become widespread, with many popular models offering hybrid powertrains. While most hybrids are designed to enhance fuel efficiency, some, like the Ford F-150 Hybrid pickup, utilize hybrid powertrains to boost electric power for towing or quicker acceleration.
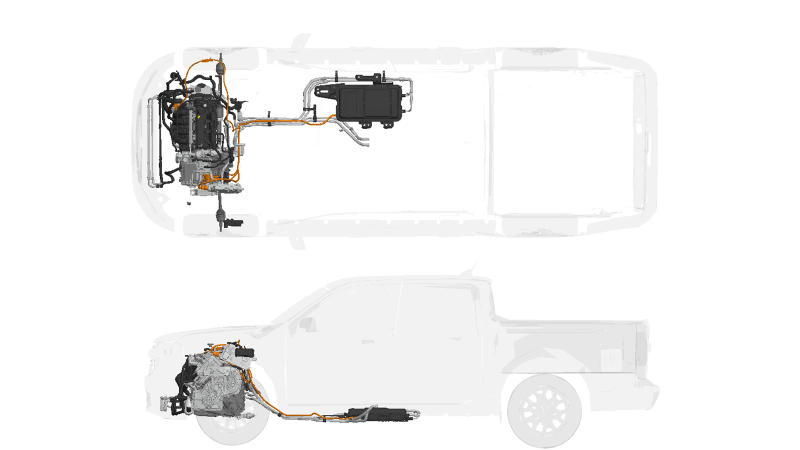
Present-day hybrids often cost the same as or less than comparable gas-powered vehicles, or they can save you money in the long run. They are typically reliable, with high owner satisfaction ratings, and often provide a better driving experience than nonhybrid versions. Moreover, hybrids do not require plugging in and can be refueled at any gas station.
Hybrid Electric Vehicles Architectures
Hybrid vehicles come in four main architectures, each with its unique characteristics and benefits.
- Parallel hybrids
- Series hybrids
- Split axle hybrids
- Power split hybrids
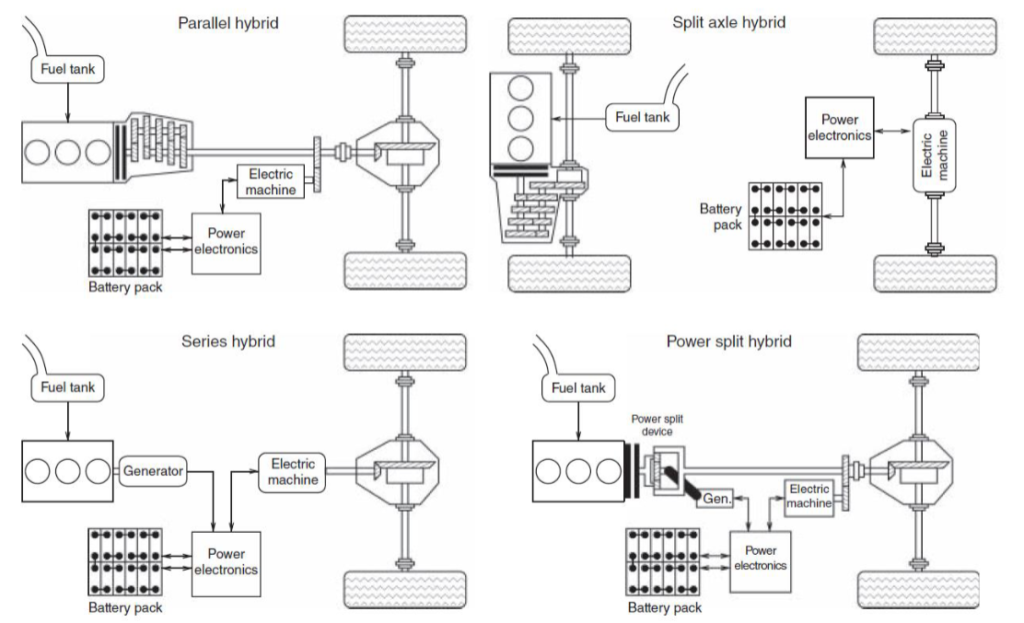
Series Hybrid Electric Vehicles
Although less common, series hybrids are gaining popularity. Similiar to other hybrids they utilize a primary drive motor to propel the vehicle using regen-collected battery energy at low speeds and harvest energy when decelerating. As speeds increase and the batteryโs energy diminishes, an engine activates to drive a second dedicated generator, converting gasoline power into electricity to replenish the battery.
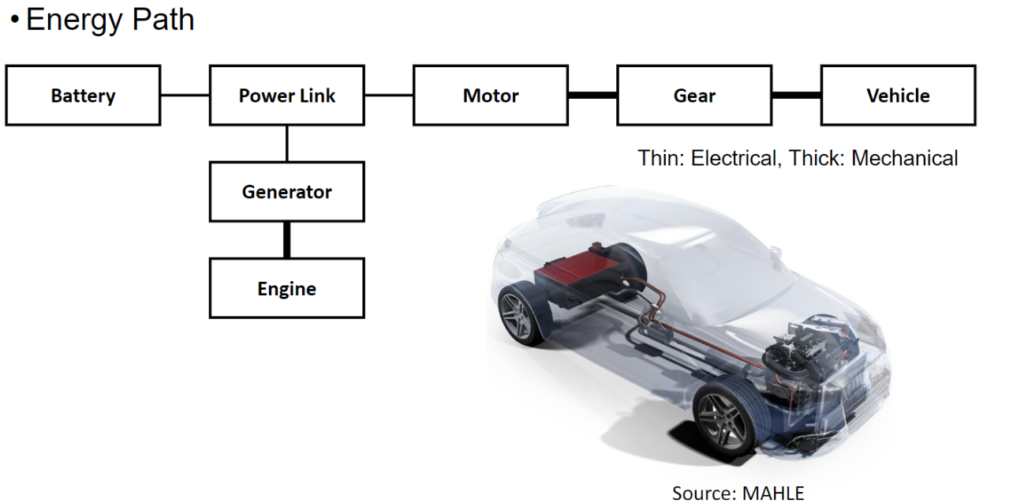
This configuration ensures that the wheels are always driven electrically, resulting in a consistently smooth and seamless power delivery. The drawback is that converting gasoline into electricity adds an extra step, reducing efficiency. However, newer approaches are mitigating this issue. Honda is a recent advocate of this hybrid type, featuring in the latest versions of the Civic, CR-V, and Accord hybrids.
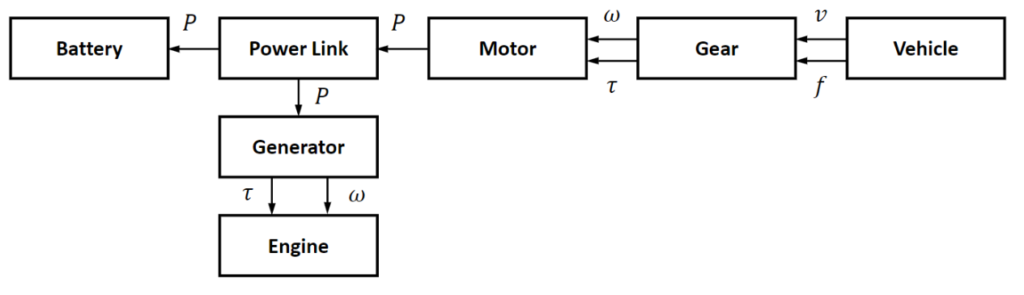
Parallel Hybrid Electric Vehicles
This is the most prevalent type oh hybrid, designed to selectively use either the gasoline engine or the electric motor to propel the vehicle. They can also utilize both power sources simultaneously. At lower speeds, the electric motor is favored due to its high initial torque and efficiency, making optimal use of the limited battery energy. Following a delayed start, the gasoline engine kicks in and gradually takes over as speed increases and stabilizes during cruising, when the engine operates most efficiently.
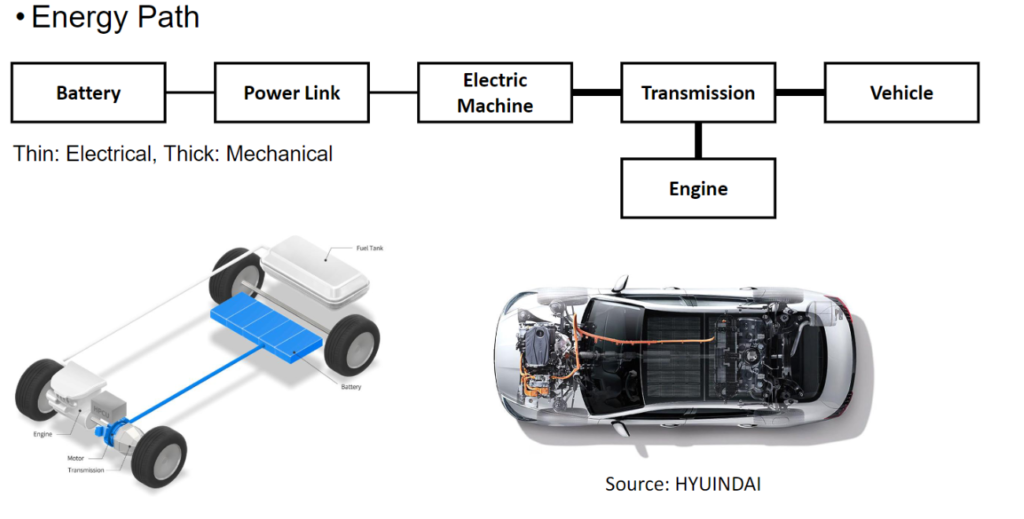
Some designs incorporate a single motor positioned between the engine and a traditional transmission, while others feature a configuration with two electric motors working in tandem to function as a continuously variable transmission for the engine. The former is exemplified by early Honda hybrids, as well as current Hyundai and Kia models like the Elantra. The latter design is employed in Toyota, Lexus, and Ford hybrids such as the Maverick.
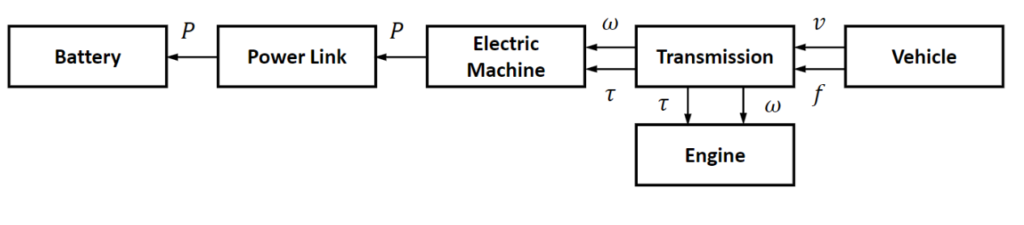
Power-split or series-parallel hybrid
Power-split hybrids, also known as series-parallel hybrids, are a type of parallel hybrid that incorporates power-split devices, enabling power paths from the internal combustion engine (ICE) to the wheels that can be either mechanical or electrical. The primary principle behind these hybrids is to separate the power supplied by the primary source from the power demanded by the driver.
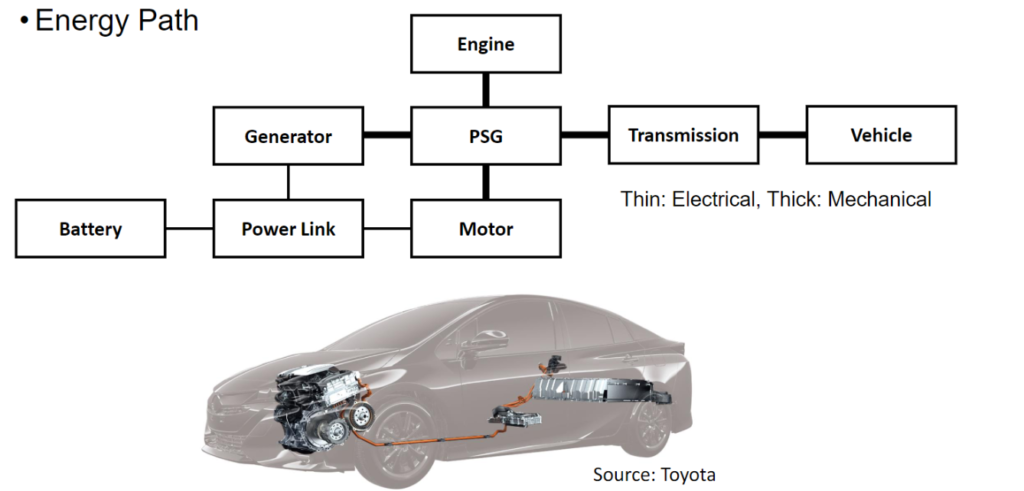
Internal combustion engines (ICEs) typically produce minimal torque at lower RPMs, leading conventional vehicles to increase engine size to meet market demands for acceptable initial acceleration. However, electric motors deliver full torque at standstill and are ideal for compensating for ICE torque deficiencies at low RPMs.
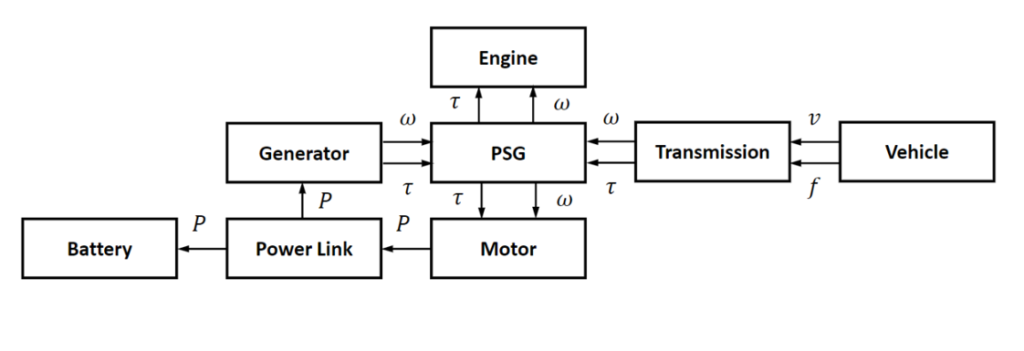
In a power-split hybrid, a smaller, less flexible, and more efficient engine can be utilized. The conventional Otto cycle, known for its higher power density and more low-RPM torque but lower fuel efficiency, is often modified to an Atkinson cycle or Miller cycle, which offer lower power density, less low-RPM torque, but higher fuel efficiency, sometimes referred to as an Atkinson-Miller cycle. This smaller, more efficient engine, operating in the favorable region of the brake specific fuel consumption (BSFC) map, significantly contributes to the vehicleโs overall higher efficiency.
Power Split Devices (PSD)
Power Split Devices (PSDs) are crucial components in hybrid vehicles, particularly in power-split hybrid architectures. In the typical configuration of a power-split HEV, a PSD connects an engine, a motor, a generator, and the drivetrain.
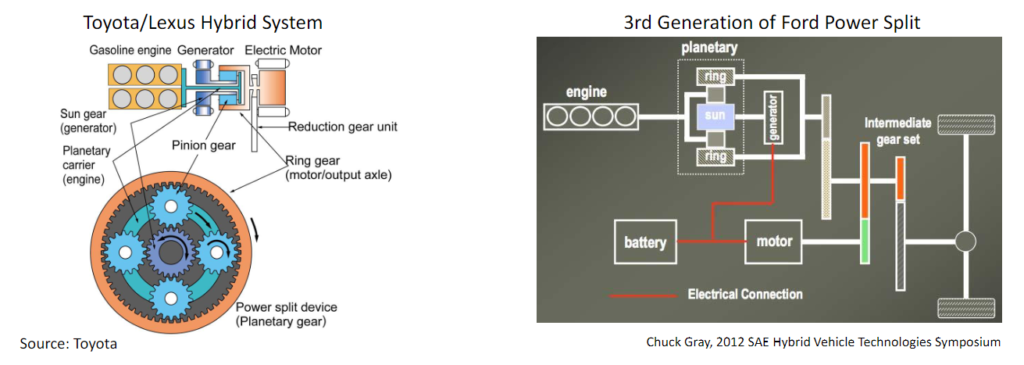
At the heart of most PSDs lies a planetary gear set, which forms the core of their functionality. These devices enable the continuous and variable mixing of power from the internal combustion engine (ICE) and the electric motor, allowing for an infinite range of gear ratios.
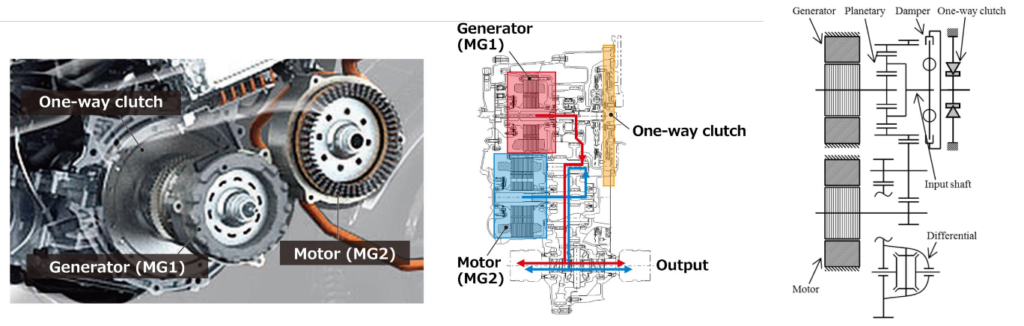
This ability to seamlessly blend power sources is what distinguishes PSDs and contributes to the efficiency and performance of hybrid vehicles, as it optimizes the use of both the engine and the electric motor in various driving conditions.
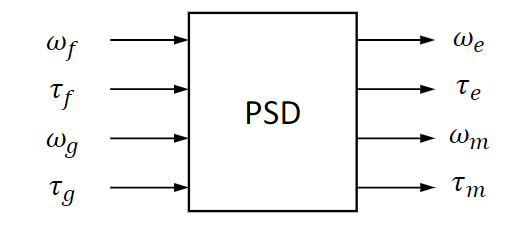
Electrified Propulsion System Explained
Electrified propulsion systems, such as those found in hybrid vehicles, follow a specific energy path to propel the vehicle. It begins with the battery, where electrical energy is stored. This energy is then sent through a power link to an electric machine, which can act as a motor to drive the vehicle or as a generator to recharge the battery.
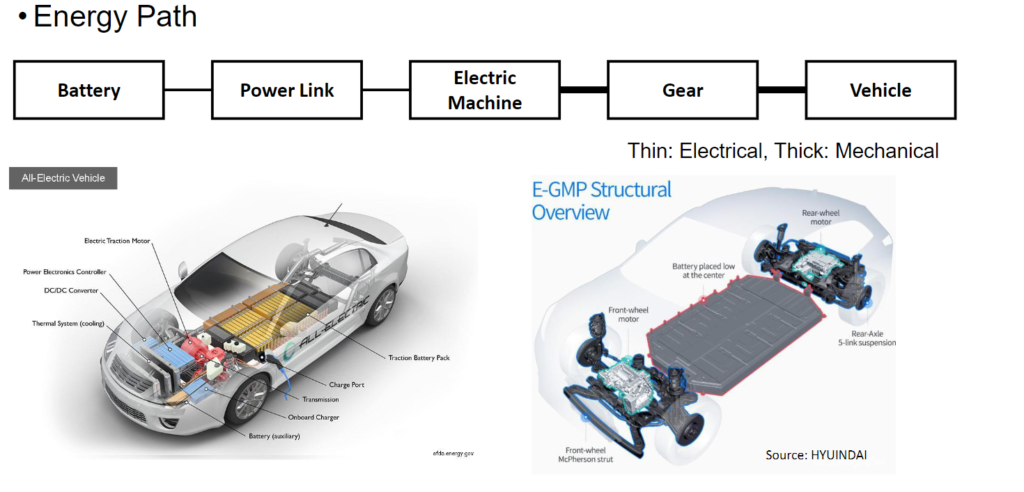
The electric machine is connected to a gear system, which helps control the speed and torque of the vehicle. Finally, the power from the electric machine is transferred to the vehicleโs wheels, either directly or through a transmission, enabling the vehicle to move efficiently. This energy path illustrates the intricate yet efficient process by which hybrid vehicles utilize electricity to reduce fuel consumption and emissions while maintaining performance.

Energy Managament/ Modeling of HEVs
Modeling of hybrid electric vehicles is essential for designing and optimizing their performance. In electrified vehicles, the electric machine plays a crucial role, serving both as a motor for propulsion and as a generator for regenerative braking. Quasistatic models are commonly used in the design and evaluation of energy management strategies for electrified vehicles. These models simplify the dynamic behavior of the vehicle and its components, allowing for the efficient analysis of different operating conditions and control strategies. By using quasistatic models, engineers can assess the performance of HEVs and optimize their energy efficiency, ultimately leading to more effective and sustainable vehicle designs.
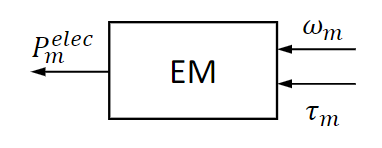
Motor Efficiency, Power/Torque Limits of HEVs
The efficiency of a hybrid electric vehicleโs motor is crucial for its overall performance and energy consumption. The relationship between electrical power ๐๐๐๐๐๐ (๐ก), mechanical power ๐๐ (๐ก) โ ๐๐ (๐ก) (torque, and speed) is described by a stationary efficiency map, denoted as ๐๐, which is a function of the torque and speed of the motor.
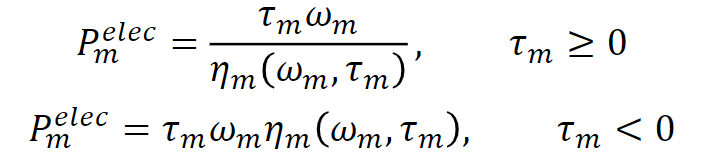
This efficiency map provides insights into how efficiently the motor converts electrical power into mechanical power. Additionally, an electrical power loss map is often used instead of an efficiency map to quantify the power lost as heat or other forms of energy.

The maximum and minimum motor torque, denoted as ๐๐,max and ๐๐,min, respectively, are crucial parameters that describe the motorโs performance limits. These torque limits are functions of the motor speed and help ensure that the motor operates within safe and efficient parameters.
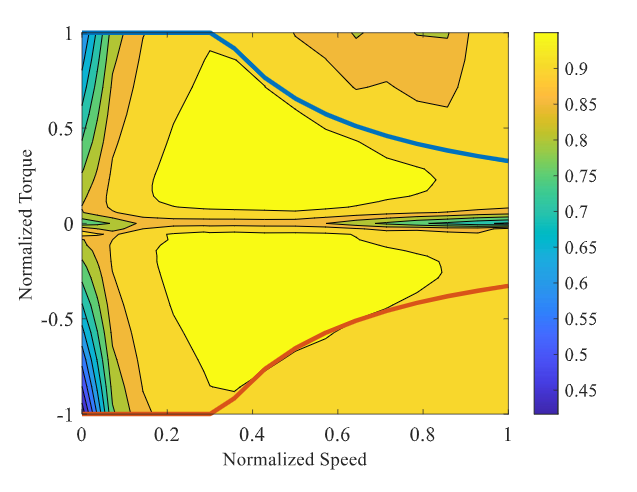
Hybrid Electric Vehicles vs. Electric Vehicles
Electric vehicles (EVs) differ from hybrids in that they lack a gasoline engine and require recharging via a plug. They can typically travel over 200 miles on a single charge and can be recharged at home or at public charging stations.
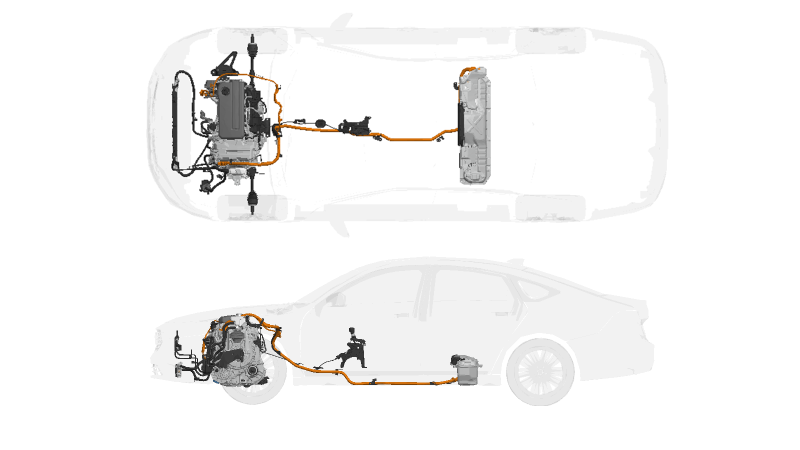
However, recharging an EV is a lengthier process compared to refueling a gasoline-powered vehicle, taking anywhere from 20 minutes to several hours depending on the charging speed and distance required. Additionally, locating a charging station while on the road is not as effortless as finding a gas station, making hybrids more convenient for individuals without easy access to EV charging stations, those unable to charge at home, or frequent long-distance travelers.
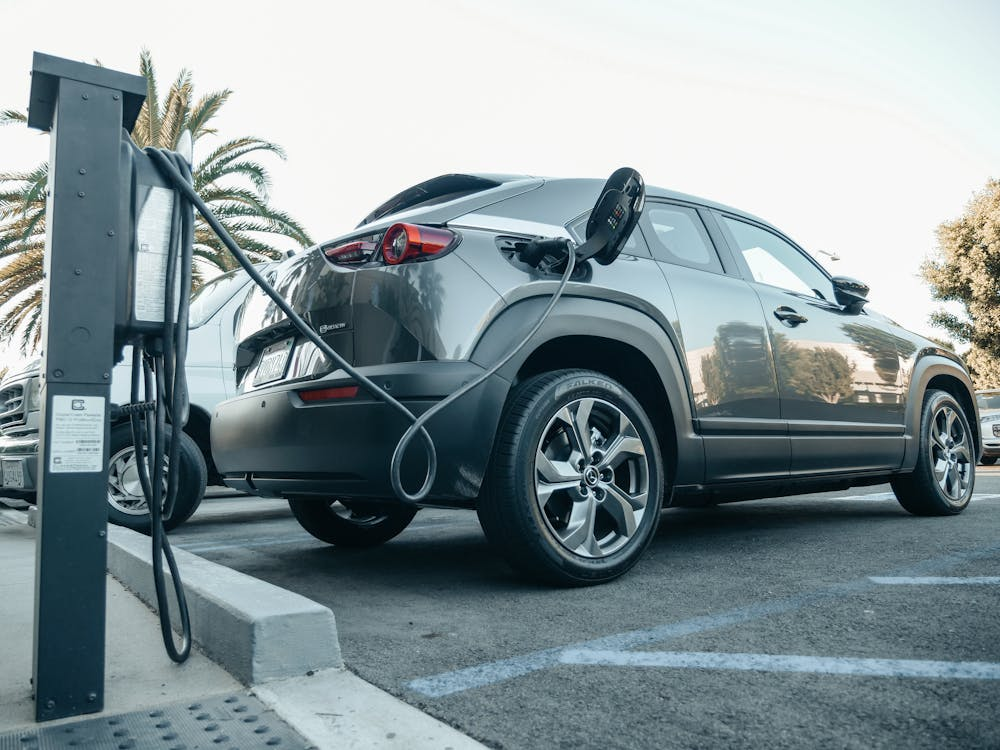
Consumer Reportsโ analysis indicates that EVs are more energy-efficient than hybrids and often incur lower maintenance and repair costs. They may also qualify for tax credits that hybrids do not. Nonetheless, in certain scenarios, a hybrid can be more cost-effective to own and operate than a comparable electric vehicle, particularly in the case of small SUVs and compact cars, particularly in regions with high electricity prices. One primary factor is their lower initial cost.
What About Plug-In Hybrids?
Plug-in hybrid electric vehicles (PHEVs) differ from pure EVs or traditional hybrids in that they operate like a hybrid but can also be plugged into a wall outlet or EV charger. When fully charged, they can primarily run on electric power for approximately 20 to 40 miles (depending on the model) before switching back to regular hybrid operation. Unlike standard hybrids, PHEVs may qualify for federal tax credits.
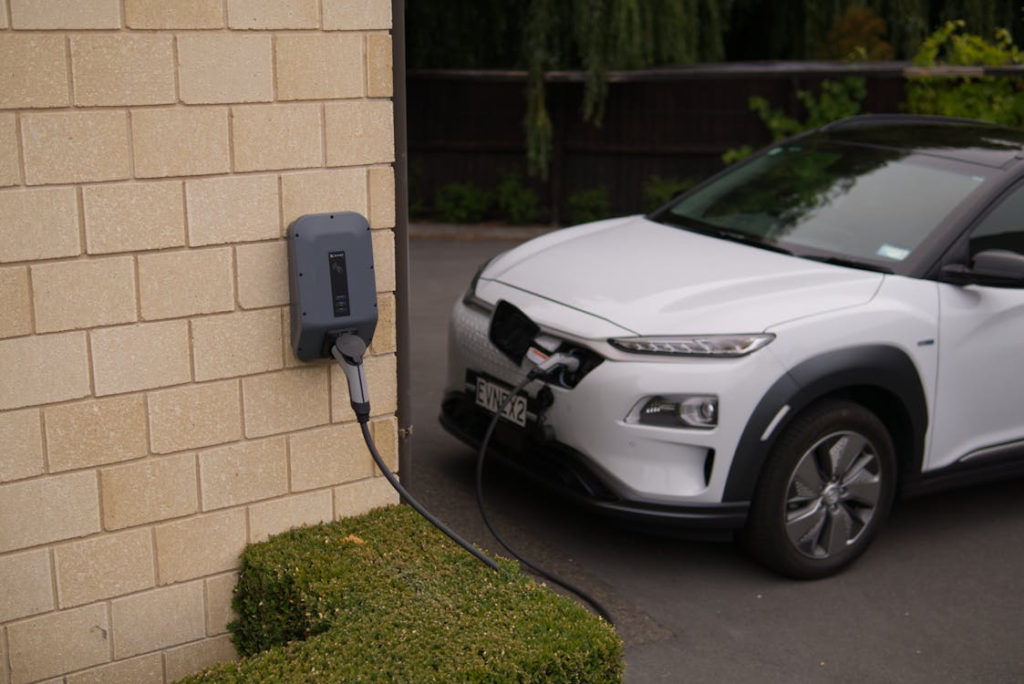
Given that the average American drives less than 40 miles per day, some drivers could complete most of their daily travel on electric power by plugging in first, reserving the gas engine for longer trips.
However, due to the additional weight of their larger batteries, motors, and onboard charging equipment, PHEVs typically cost more and achieve lower mileage than conventional hybrids once the electric charge is depleted. If you charge the vehicle daily and have a short commute, your fuel consumption would be minimal. However, in other scenarios, your fuel consumption could exceed that of a regular hybrid. If you can charge at home and rarely embark on long journeys, a pure EV might be a more suitable option.
Components of a Hybrid Electric Vehicle
- Auxiliary Battery: This low-voltage battery provides initial power to start the car and operates vehicle accessories before the main traction battery is engaged.
- DC/DC Converter: Converts higher-voltage DC power from the traction battery pack into the lower-voltage DC power required to run vehicle accessories and recharge the auxiliary battery.
- Electric Generator: Generates electricity by harnessing energy from the rotating wheels during braking, transferring it back to the traction battery pack. Some vehicles use motor generators that serve both propulsion and regeneration functions.
- Electric Traction Motor: Drives the vehicleโs wheels using power from the traction battery pack. Some vehicles feature motor generators that handle both propulsion and regeneration duties.
- Exhaust System: Channels exhaust gases from the engine out through the tailpipe. A three-way catalyst within the system reduces engine emissions.
- Fuel Filler: Receives the nozzle from a fuel dispenser to fill the gasoline tank.
- Gasoline Tank: Stores gasoline until itโs needed by the engine.
- Spark-Ignited Internal Combustion Engine: Injects fuel into either the intake manifold or the combustion chamber, where it mixes with air and is ignited by a spark plug.
- Power Electronics Controller: Manages the flow of electrical energy from the traction battery, regulating the speed and torque output of the electric traction motor.
- Cooling Thermal System: Maintains proper operating temperatures for the engine, electric motor, power electronics, and other components.
- Traction Battery Pack: Stores electricity for use by the electric traction motor.
- Transmission: Transfers mechanical power from the engine and/or electric traction motor to drive the vehicleโs wheels.
How Hybrids Benefit You
One of the primary advantages of any hybrid vehicle is its ability to capture and reuse braking energy that would otherwise be dissipated as heat and wear on the brakes. The hybrids that attract the most interest utilize this recovered energy to save fuel and improve miles per gallon (MPG) by employing an electric motor to delay the activation of the gasoline engine. However, performance-oriented hybrids utilize the harvested energy to enhance overall power and speed.
Fuel-saving hybrids are an appealing option for individuals seeking to save money or reduce their carbon footprint. After all, high MPG and low carbon emissions go hand in hand. For those living in apartments or requiring only one vehicle, an electric vehicle (EV) might be impractical. In contrast, a hybrid is a gasoline-fueled vehicle that does not have the constraints or special refueling needs of an EV.
From a practical standpoint, a hybridโs emphasis on regenerative braking results in significantly longer-lasting brake pads and rotors compared to conventional vehicles. Additionally, hybrids do not require a separate starter motor, as this function is handled by the electric motor. Furthermore, the small size of a hybridโs battery means it takes up minimal space and does not necessitate expensive battery chemistry.
Conclusion
In conclusion, hybrid electric vehicles (HEVs) represent a significant step forward in automotive technology, offering a blend of fuel efficiency, reduced emissions, and performance. By incorporating both an internal combustion engine (ICE) and an electric motor, HEVs can optimize power delivery based on driving conditions, resulting in improved fuel economy compared to traditional vehicles.
The ability to capture and reuse braking energy through regenerative braking further enhances their efficiency. While there are different architectures of HEVs, each with its unique characteristics, they all contribute to a greener and more sustainable future for transportation. As technology continues to advance, HEVs are expected to play a key role in the transition towards fully electric vehicles, offering a practical and efficient alternative for drivers around the world.