Automotive Aerodynamics 101
Have you ever wondered why some cars are faster and more efficient than others? Well, it all comes down to something called automotive aerodynamics. The field of automotive aerodynamics involves examining the airflow around a vehicle. The goal is to diminish drag and wind noise and avoid unintended lift forces contributing to aerodynamic instability, particularly at high speeds. With sports cars/ race cars, vehicle aerodynamics are used to generate downforce to enhance traction and thereby improve cornering performance. In this article, I’m going to explain the basics of automotive aerodynamics and how it can improve a car’s fuel efficiency and performance.
When did engineers start studying automotive aerodynamics?
As vehicles started to become faster engineers started noticing that aerodynamic drag escalates notably with vehicle velocity. So in the 1920s, engineers began studying how to use an automobile shape to diminish aerodynamic drag at higher speeds. By the 1950s, German and British automotive engineers systematically examined the impacts of automotive drag on higher-performance vehicles. By the late 1960s, scientists also recognized the rise in aerodynamic noise emitted by automobiles when traveling at high speeds.
What are the basics of automotive aerodynamics?
So, what is automotive aerodynamics? It’s the study of how air flows around a car and how that affects its performance. There are two types of airflow: laminar and turbulent. Laminar airflow is smooth and predictable, while turbulent airflow is chaotic and unpredictable.
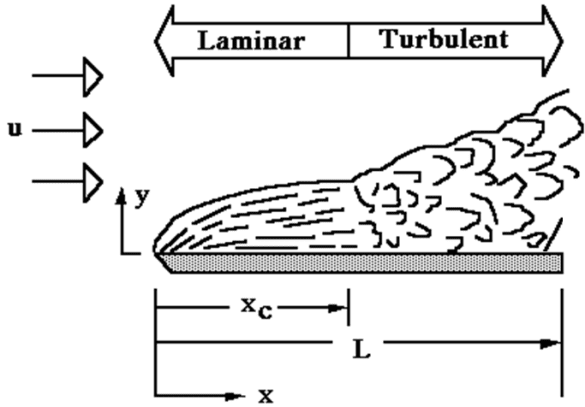
How are car aerodynamics tested?
Automotive Aerodynamics testing is performed with a combination of physical testing at a wind tunnel and computational fluid dynamic software. The more streamlined a vehicle is, the greater its efficiency leading to an improved range or fuel economy.
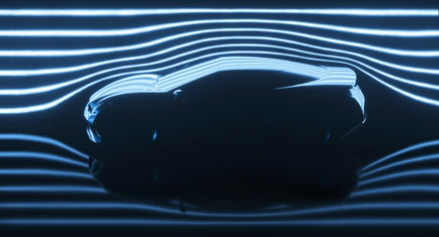
An aerodynamic wind tunnel typically comprises a test section where a vehicle can be mounted primarily on the pinch welds on the underbody subassembly. Air is either blown or usually sucked over the vehicle by a fan. To ensure the accuracy of the results the tires are spun during the test. This ensures the drag generated by the moving tires is captured in the wind tunnel testing. Most of the airflow that a vehicle will encounter will be turbulent. Turbulent air is extremely high-energy airflow, which makes it difficult to predict.
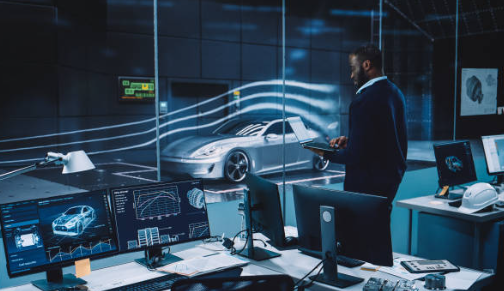
What are the three aerodynamic forces?
let’s talk about the three aerodynamic forces: lift, drag, and side forces. Lift is the force that allows an airplane to stay in the air, while drag is the force that slows down a car. Side forces are the forces that push a car to the side.
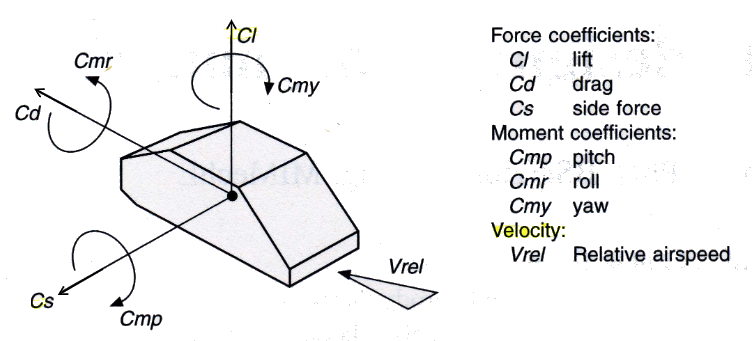
It is important to remember: 𝑣 = 𝑣𝑣𝑒ℎ − (𝑣𝑤𝑖𝑛𝑑) 𝑣𝑤𝑖𝑛𝑑: wind is (-) during headwind conditions.
What is Drag in Automotive Aerodynamics:
Aerodynamic drag is the force that resists the forward motion of a car. It is a force that acts along the direction of the moving body, opposing its motion through the air. As a car moves forward, it encounters air resistance, caused by the air pushing against the front, sides, and rear. This resistance increases with the speed of the car and is a key factor in determining fuel efficiency and performance. Car designers strive to reduce aerodynamic drag to minimize air resistance, thus improving fuel efficiency and overall performance.
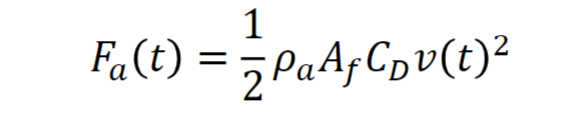
Aerodynamic drag force depends on Frontal Area 𝐴𝑓, drag coefficient 𝐶𝐷, air density 𝜌𝑎, and vehicle velocity 𝑣.
Approximate contributions to 𝐹𝑎 in an ICE vehicle:
- 65% car body
- 20% wheel housings
- 10% exterior mirrors, window housings, etc.
- 5% engine ventilation
What is Lift in Automotive Aerodynamics:
Aerodynamic lift is a vertical force that is perpendicular to the direction of aerodynamic drag. While drag resists the forward motion of a car, lift acts either upwards or downwards (downforce), depending on the design and orientation of the car’s body. Lift is typically associated with the force that lifts the front of the car at high speeds, potentially affecting stability and handling. Car designers carefully consider and balance lift and drag forces to optimize the aerodynamic performance of a vehicle, ensuring it remains stable and efficient across a range of speeds.
What is Side Force in Automotive Aerodynamics:
Aerodynamic side force is a lateral force that occurs in crosswind conditions or when a vehicle is near another object, such as another vehicle or a barrier. Think of how the 18-wheeler moves your car when driving down the interstate. This force acts perpendicular to the direction of motion, pushing the vehicle sideways. Side force can affect the stability and handling of a vehicle, especially at high speeds.
Automotive Aerodynamics Force and Moment equations:
The aerodynamic force and moment equations are used to calculate the forces and moments acting on an object moving through air. These equations relate the aerodynamic forces (lift, drag, and side force) and moments (torques) to the properties of the fluid and the geometry of the object.
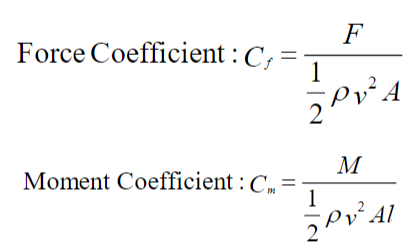
F = force (lift, drag, side); M = moment; A = reference area; l = reference length; v= velocity; p= air density
What are the different types of drag on a car?
Different types of drag affect a car’s performance, such as pressure drag, skin friction drag, and form drag. To improve a car’s performance, we need to reduce drag. This can be done by improving the car’s shape, reducing its frontal area, and using smooth surfaces.
Form Drag / Pressure Drag in Automotive Aerodynamics:
Form drag, also known as pressure drag, is a significant component of aerodynamic drag in automotive design. It is caused by the motion of air around the vehicle, which leads to changes in air pressure. As the vehicle moves, the shape and frontal area of the vehicle cause the air to either compress or expand. This results in pressure differences that create drag.
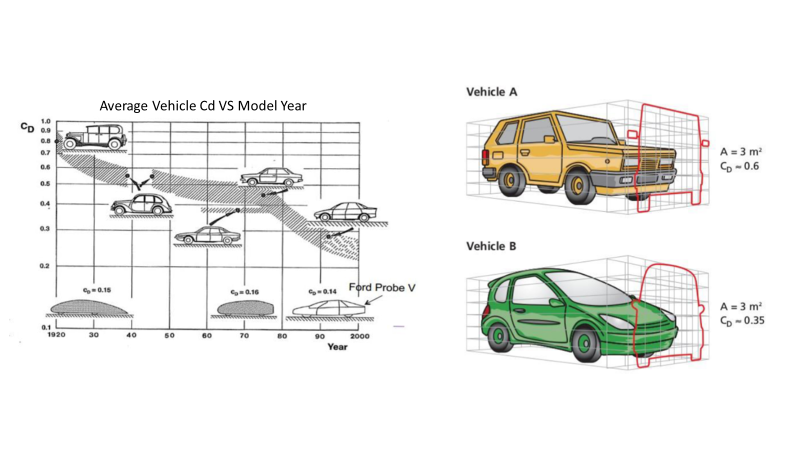
Form drag is the most significant drag force affecting a moving vehicle. It impacts overall vehicle efficiency and increases with vehicle speed. Therefore, car designers focus on optimizing the external shape and frontal area of the vehicle.
Surface Drag / Skin friction Drag in Automotive Aerodynamics:
Surface drag, also known as skin friction drag, is another important component of aerodynamic drag in automotive design. It is caused by frictional forces between the body of the vehicle and the air flowing over it. As air flows over the surface of the vehicle, it creates a thin layer of air near the surface called the boundary layer.
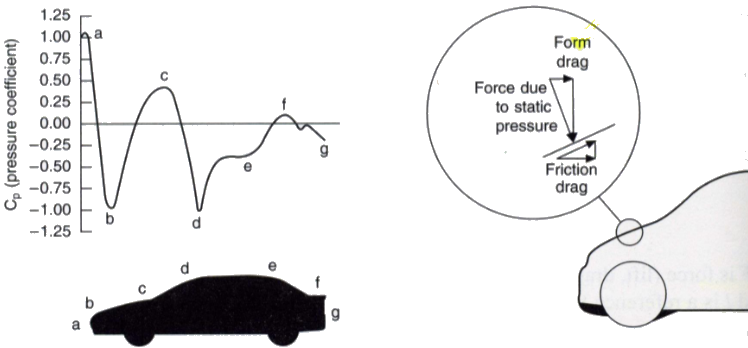
The frictional forces at the boundary layer result in skin friction drag. The magnitude of skin friction drag depends on the shear stress at the boundary layer, which is influenced by factors such as the surface roughness of the vehicle and the viscosity of the air. Car designers aim to reduce skin friction drag by smoothing the surface of the vehicle and minimizing surface roughness, thereby improving the overall aerodynamic efficiency of the vehicle.
Excrescence drag Explained:
Excrescence drag is a type of aerodynamic drag that occurs due to components on a vehicle that disturb the airflow and create turbulence. These components include both front and rear wheels, side mirrors, door handles, rain gutters, windshield wiper blades, and exhaust systems. While these components are necessary for the functioning of a vehicle, they also increase drag and wind noise, reducing fuel efficiency and overall performance. Car manufacturers often design these components to minimize their impact on aerodynamic efficiency, such as by optimizing their shape and placement to reduce turbulence and drag.
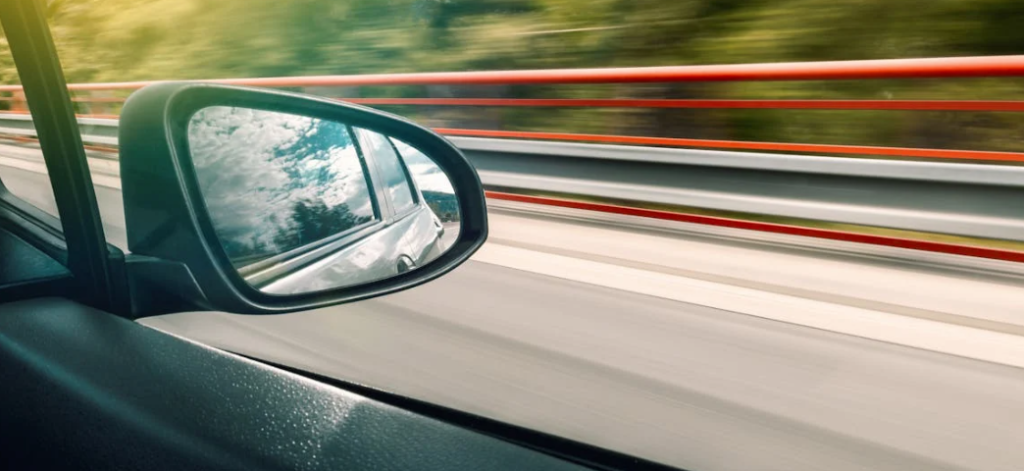
What is Internal drag:
Internal drag in a vehicle refers to aerodynamic drag that arises from airflow within the vehicle itself. This type of drag is caused by the cooling of the engine in an internal combustion engine (ICE) vehicle or the battery in an electric vehicle (EV), as well as the cooling of other mechanical components such as brakes.
Additionally, airflow from cabin ventilation systems can contribute to internal drag. Managing internal drag is important for vehicle efficiency and comfort, as it affects how efficiently the vehicle operates and how comfortable passengers are inside the vehicle. Car manufacturers work to design efficient cooling systems and ventilation systems to minimize internal drag and improve overall vehicle performance.
How can drag be reduced?
Drag can be reduced through various aerodynamic design strategies. One common approach is to streamline the vehicle’s shape to minimize air resistance. This includes reducing sharp edges, smoothing out contours, and tapering the rear of the vehicle. Another method is to minimize the vehicle’s frontal area, as a smaller frontal area reduces the amount of air the vehicle needs to push through.
Other techniques include adding features like air dams, diffusers, and spoilers to manage airflow around the vehicle more effectively. Additionally, improving the surface quality of the vehicle to reduce skin friction drag and optimizing the design of components that protrude from the vehicle, such as side mirrors and door handles, can also help reduce drag. Overall, reducing drag is crucial for improving fuel efficiency and overall vehicle performance.
How can I reduce the drag on my car?
To reduce drag on your current vehicle, you can follow several strategies. Firstly, driving with the windows up can help maintain smoother airflow around the vehicle, reducing drag. Secondly, switching to low-rolling resistance tires can decrease the drag generated by more aggressive tire designs (off-road tires) reducing energy loss and improving fuel efficiency.
Additionally, switching to aerodynamic wheels with smaller openings between spokes can help reduce drag by allowing air to flow more smoothly around the wheels. Another approach is to adjust the front fascia and air dam to reduce drag under the vehicle, as well as install side skirts to cover the underside of the vehicle and further reduce drag. Lastly, reducing the number of protrusions on the surface of the car, such as removing roof racks or large side mirrors, can also help minimize drag and improve aerodynamics.
Drag Reduction and Vehicle Dynamics:
Dynamic stability is an essential consideration in vehicle design, especially for low-drag designs. While low-drag designs are efficient for reducing fuel consumption and improving performance, they can be more sensitive to external factors such as cross-winds, side forces, and yawing moments.
Enabing drag reduction: Front Facia :
The front end of a car plays a crucial role in aerodynamic drag reduction. To achieve this, engineers often employ various strategies. One common approach is to lower the front fascia or add an air dam to reduce the amount of air that flows underneath the vehicle, as airflow under the car can increase drag. Rounding off the sharp front corners of the front fascia is another effective method to reduce the vehicle’s drag coefficient, as sharp edges can create turbulence.
What does an Air Curtain do?
However, in cases where rounding off the front corner is not feasible due to styling concerns, engineers may opt to add air curtains to the vehicle design. An air curtain is a vent located at the front corners of a vehicle, enabling air to pass beneath the headlamps, into the wheel housings, and around the front wheels.
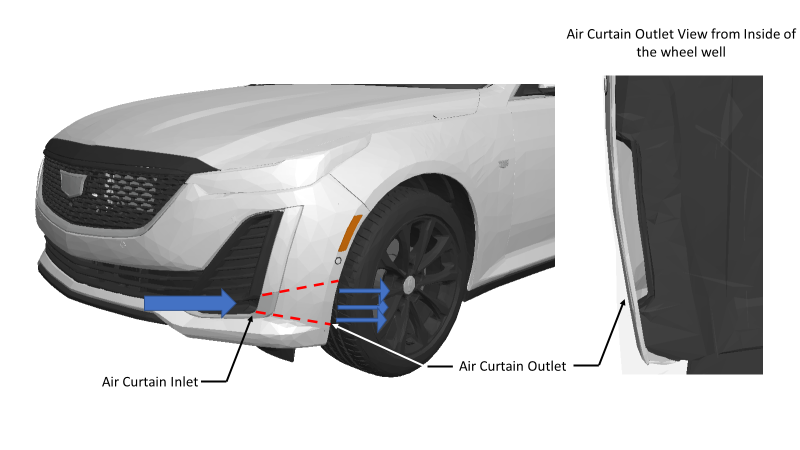
This design directs airflow in a manner that effectively reduces the air resistance of the vehicle body in a straightforward yet highly efficient manner. These design considerations are crucial in achieving optimal aerodynamic performance and improving the overall efficiency of the vehicle.
How Windshield Angle Affects Drag:
The angle of a windshield ( SAE A121-1) can significantly impact a vehicle’s drag. Increasing the windshield angle can help reduce overall vehicle drag, as it promotes smoother airflow over the vehicle. However, this design choice comes with drawbacks. One major drawback is the reduction in cabin space and driver headroom inside the vehicle, as the increased angle may encroach on interior space. Another issue is optical reflection and poor light transmission, which can occur as a result of the increased angle.
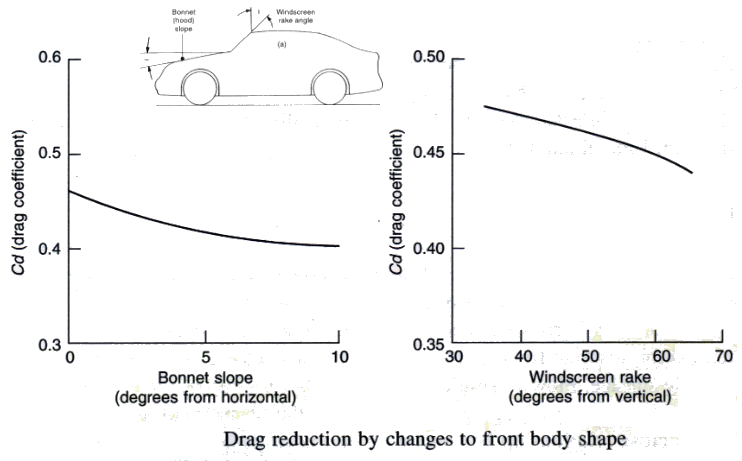
The relationship between Lift and drag:
The relationship between lift and drag in vehicle aerodynamics is crucial for understanding the forces that affect a vehicle’s performance. The creation of a profile for passenger space and mechanical design requirements often induces a vertical component force known as lift. This lift, in turn, induces changes in the flow of air around the vehicle, creating an induced drag force.
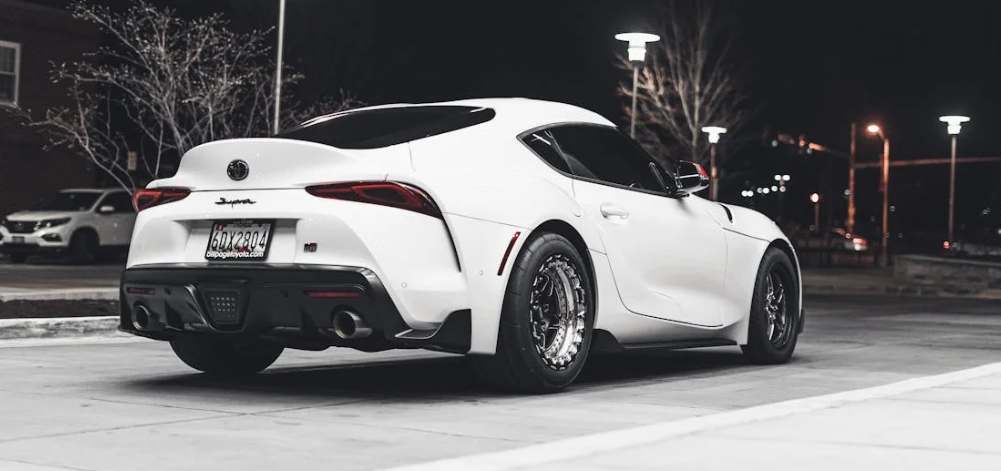
To counteract lift and improve stability, sports cars, and race cars designed to travel at higher speeds often incorporate a rear wing/ spoiler. A rear spoiler is designed that extends above the rear deck lid to enhance downforce, decreasing lift at high speeds but also resulting in increased drag. Automotive engineers take the drag penalty to increase downforce, pressing the vehicle to the ground and providing additional stability to the driver, especially during high-speed maneuvers.
How the airflow over the rear surfaces affects Automotive Aerodynamics:
The airflow over the rear surfaces of a vehicle plays a critical role in automotive aerodynamics. A square back design, commonly found in traditional sedan cars or a boxy full-size SUV, tends to create a large, low-pressure wake behind the vehicle. This wake results in increased aerodynamic drag, reducing fuel efficiency. On the other hand, a hatchback design, characterized by a more sloping rear, can lead to vortex generation.
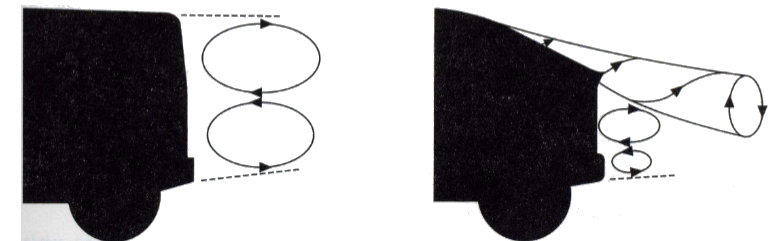
These vortices can help energize the airflow, reducing the size of the wake and potentially decreasing aerodynamic drag. Engineers carefully consider the rear surface design of vehicles to optimize aerodynamics, and balancing factors such as drag reduction and aesthetic appeal.
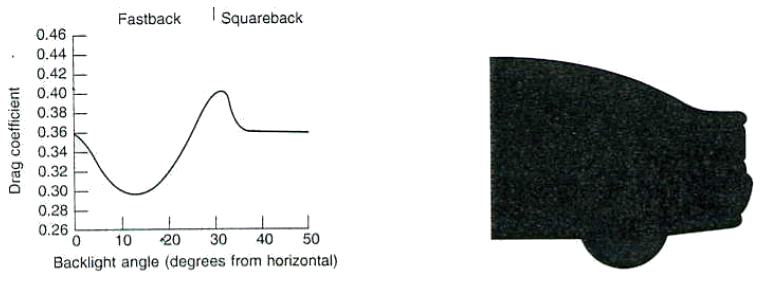
How the Underbody affects a vehicles drag:
Reducing drag on the underbody of a vehicle is commonly done using aerodynamic features such as underbody panels. The smoother the underbody the lower the drag. An example of this is the 2020 Porsche Taycan 4S Performance Plus underbody. The underbody on the Taycane was designed to reduce turbulent airflow. The black underbody covers are placed below the front and rear bumper fascia, rockers, and suspension components. Trade-offs between aero drag vs additional panels (costs, vehicle mass).
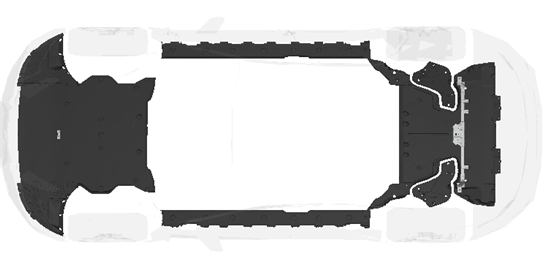
What is the optimum angle for a diffuser?
The diffuser angle, which is related to the departure angle of a vehicle, can significantly impact its drag coefficient. The diffuser is a shaped section of the rear of the vehicle that helps manage the airflow underneath the car.
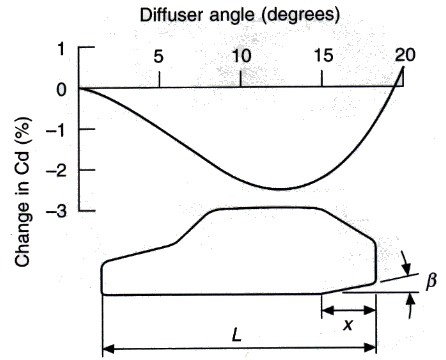
Car designers carefully optimize the diffuser angle to achieve the best balance between aerodynamic efficiency and other design considerations. Adjusting the diffuser angle between 9.8° – 16° eliminates positive pressure disturbances at the underbody edge, indicating that this angle is optimal for the diffuser.
Influence of Body Shape on Automotive Aerodynamics
The body shape of a car also plays a big role in its aerodynamics. Car manufacturers design car bodies to optimize aerodynamics, such as by using a teardrop shape to reduce drag. This is why some cars look sleeker than others.
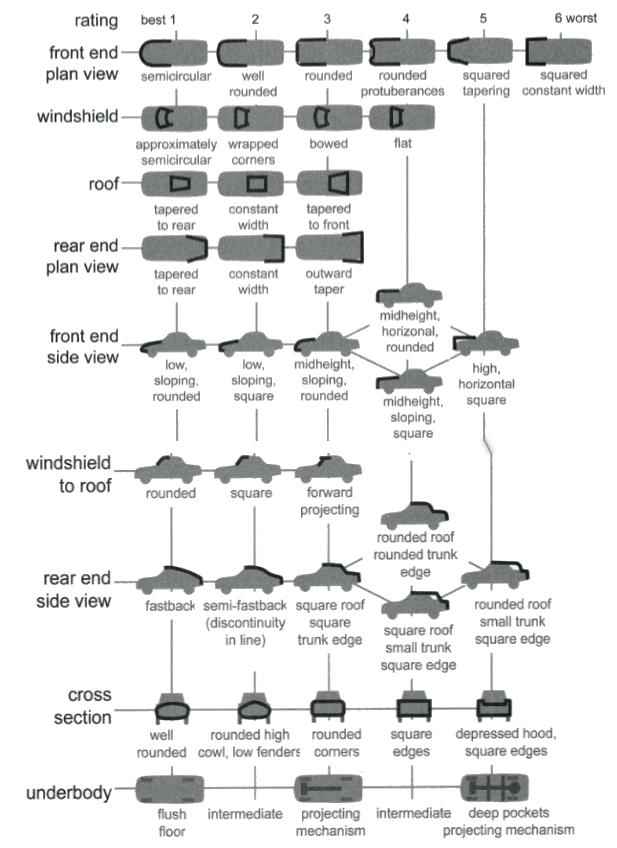
How Automotive Aerodynamics Affects Stability and Cross-winds
Automotive aerodynamics significantly impacts the stability of passenger cars, with two main concerns being the “feel” of the car at high speeds and its lane maneuverability. The feel of a car traveling in a straight line at high speed and in calm conditions is crucial for driver confidence and comfort. However, quantifying this aspect of stability can be challenging. Additionally, lane change stability is affected by increases in overall lift and differences in lift between the front and rear axles. Designing a vehicle with balanced aerodynamics can help maintain stability during lane changes and high-speed driving/ maneuverability.
Another important consideration is the effect of cross-winds on vehicle stability. Steady cross-winds, while not a safety issue, can impact the vehicle’s aerodynamic drag and create additional wind noise. On the other hand, transient gusts pose a more significant challenge. To address this, vehicles are designed to minimize the side forces and yawing moments caused by transient cross-winds. By optimizing the vehicle’s aerodynamic profile, engineers can enhance stability and improve the driving experience in various conditions.
Automotive Aerodynamics and Ventilation
Automotive aerodynamics plays a significant role in vehicle ventilation systems. Underhood ventilation is essential for cooling the engine and other mechanical components, ensuring they operate efficiently. Efficient underhood ventilation helps maintain optimal engine temperatures and prevents overheating. Cabin ventilation, on the other hand, ensures a comfortable interior environment for passengers by providing fresh air and removing stale air. The design of ventilation systems is influenced by aerodynamics to ensure efficient airflow both under the hood and inside the cabin, contributing to overall vehicle performance and passenger comfort.
Underhood ventilation (Front end airflow):
Underhood ventilation, particularly for internal combustion engine (ICE) vehicles, is crucial for maintaining engine temperature and overall performance. The engine cooling/ air intake system, is responsible for 10-15% of a car’s drag. Airflow through the front end of the vehicle helps dissipate heat generated by the engine and other components. Proper underhood ventilation ensures that the engine operates within the optimal temperature range, enhancing efficiency and longevity.
Underhood ventilation serves another critical function: supplying air to the vehicle’s air intake system. This airflow is essential for the engine to receive the necessary oxygen for combustion. Without an adequate amount of air intake, the engine cannot ignite the fuel, leading to a loss of power or complete engine failure. However, larger openings in the front fascia for increased airflow can also have a significant impact on aerodynamic drag. Therefore, designers must carefully balance the need for proper ventilation with the desire to minimize drag for optimal vehicle performance.
Why are electric cars more aerodynamic?
One of the reasons electric vehicles (EVs) are typically more aerodynamic than internal combustion engine (ICE) vehicles is that they require less airflow for cooling. This is because cooling a battery requires less air than cooling an engine, which also uses some of the airflow to support the combustion process. This allows EVs to have much smaller grille openings, enabling sleeker front-end designs that reduce drag and improve energy efficiency.
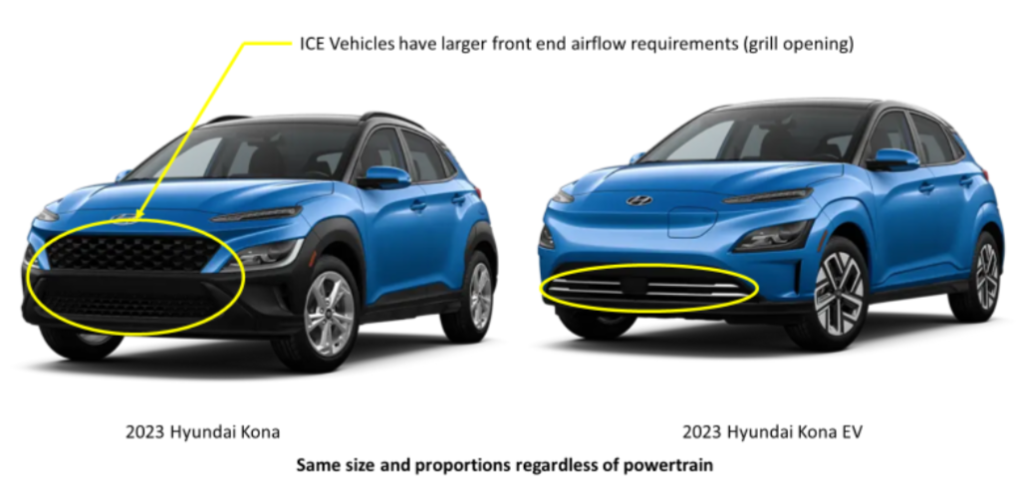
Cabin ventilation:
Cabin ventilation is crucial for maintaining a comfortable interior environment for passengers. The intake for cabin ventilation is typically located in a high-pressure zone, such as the region immediately ahead of the windshield. Placing the intake here helps ensure a supply of fresh air while minimizing the intake of dust and debris from the road surface.
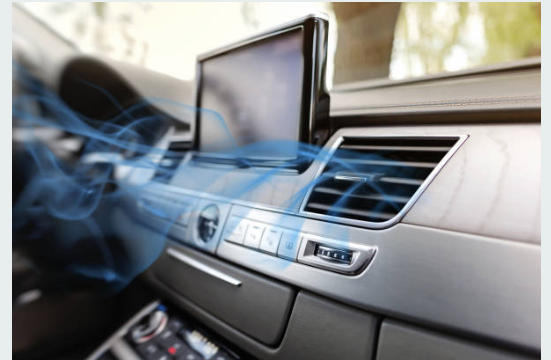
On the other hand, the extraction point for cabin ventilation is usually in a lower-pressure zone, such as the rear of the vehicle. This location allows for the efficient removal of stale air from the cabin, helping to regulate temperature and humidity levels. By carefully designing the intake and extraction points, automakers can create an effective cabin ventilation system that enhances passenger comfort.
Conclusion
In conclusion, understanding the basics of automotive aerodynamics is essential for appreciating its impact on vehicle design, performance, and efficiency. From reducing drag and improving fuel economy to enhancing stability and minimizing wind noise, aerodynamics plays a crucial role in shaping the modern automobile.
By optimizing aerodynamic design, manufacturers can create vehicles that are not only more efficient and environmentally friendly but also safer and more comfortable to drive. As technology advances and innovations emerge, the study of automotive aerodynamics continues to evolve, shaping the future of the automotive industry.