Second Life Batteries
Abstract
The Li-ion Second Life Batteries application is of growing interest with significant potential to address sustainability and environmental challenges. By 2040, an estimated 3.4 million kilograms of LIB cells from electric vehicles (EVs) could potentially enter the waste stream. However, these batteries still have many useful years ahead of them. Instead of being trashed, they can be remanufactured to be used in less demanding applications.
Benefits & Challanges
Thus extending their life beyond their original automotive application. Waste management assessments have indicated that reusing LIBs for second life applications is one of the most promising approaches to enhancing sustainability in the energy industry. However, this practice presents its own challenges, particularly in terms of safety, efficiency, and economic viability. The remanufacturing process is complex, problematic, and hazardous, requiring careful handling and specialized expertise.
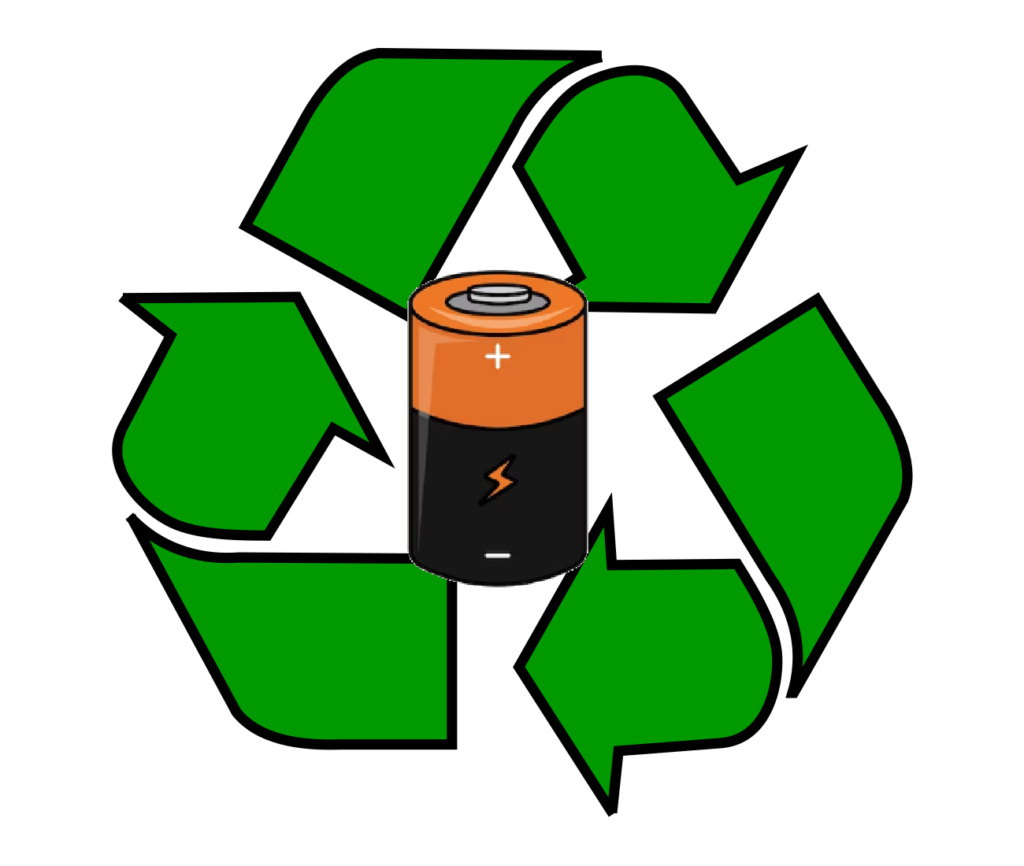
Real World Application
However, even with these challenges, LIB Second Life Application is a field with considerable potential, drawing interest from major OEMs like Nissan, Volvo, Audi, and BMW. These large OEMs have already begun experimenting with reuse/ recycling methods for these batteries. The biggest benefit for Second life batteries (SLB) comes when partnered up with renewable energy sources. Since renewable energy tends to be unstable, utilizing SLB as an energy storage system offers a promising solution. Thanks to their scalability, flexibility, fast dynamics, and, most importantly, low cost. While this area requires further study, the future of second-life applications for LIBs appears promising and could play a significant role in our transition to a cleaner future.
Future research
Research indicates that by the year 2040, approximately 3.4 million kg of LIB cells from EVs could enter the residual disposal stream [1]. Recycling LIBs involves several hurdles, including material complexity and high processing costs. LIBs contain many important metals like lithium, nickel, and cobalt. The process of extracting and separating these metals is costly and consumes a significant amount of energy. It also releases harmful emissions if not carried out in a controlled environment.
The Rise of Electric Vehicles
In recent years the electric vehicle (EVs) market has begun to expand rapidly as countries and consumers prioritize sustainable transportation options. Because of their high energy density and long lifespan, lithium-ion batteries (LIB), power the great majority of modern EVs. However, as EV adoption grows, worries regarding the environmental impact of battery disposal surface.
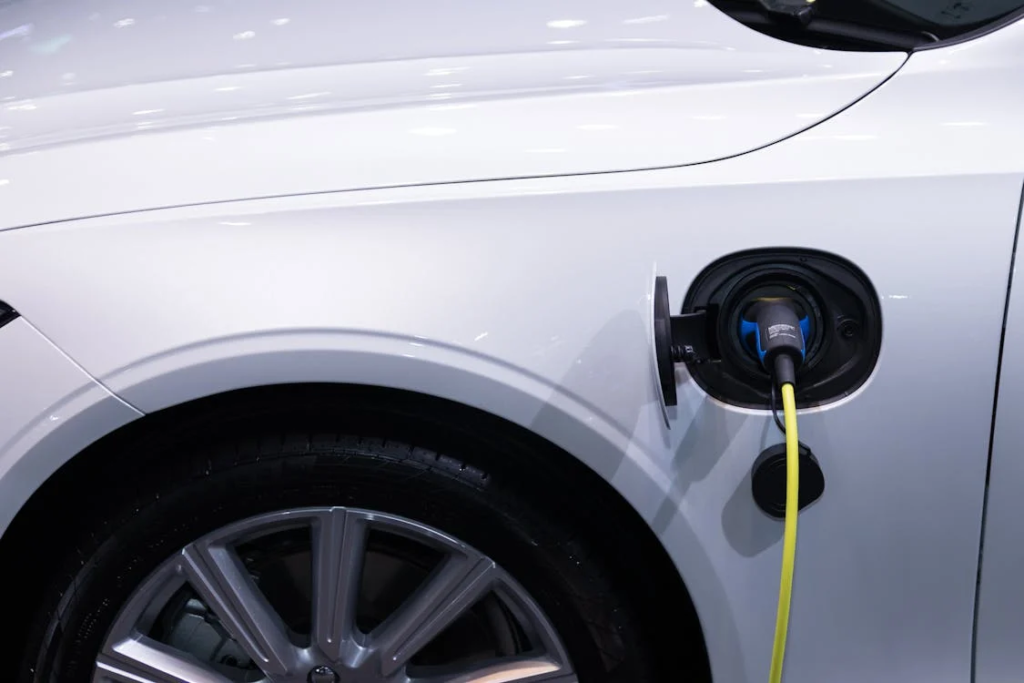
What are Second Life Batteries
This is where the idea of second life batteries comes into play. When an EV battery capacity goes below 70% to 80%, several manufacturers recommend replacing the batteries. This is due to the significant loss of range. When the battery pack can no longer properly meet operational demands, it is usually removed from the car. This means the end of its automotive life, but not necessarily the end of its utility. Repurposing retired batteries for second-life applications can assist in reducing the environmental impact of disposal while also increasing the value and utility of these valuable energy storage devices.
How long do second life batteries last?
Estimating the lifespan of second life batteries for stationary applications reveals a range from 30 to 6 years, varying according to the specific application. The battery’s second life begins when the capacity falls between 80% and 30%. During this time, they can still be employed in various applications and industries where the demands on the batteries are less strict than in an EV.
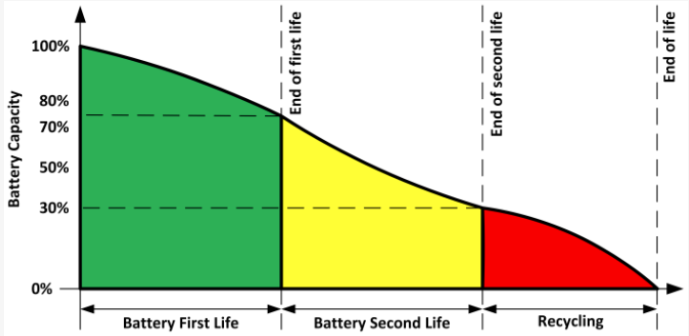
Some examples include home and commercial energy storage, offering backup power for renewable energy sources like solar and wind farms. They can also be utilized in off-grid power systems, EV charging infrastructure, EV Battery retrofitting, where some companies employ old, retrofitted batteries in older EV models to enhance the range and performance, and many other applications. Finally, when the capacity drops below 30%, LIBs are sent for recycling.
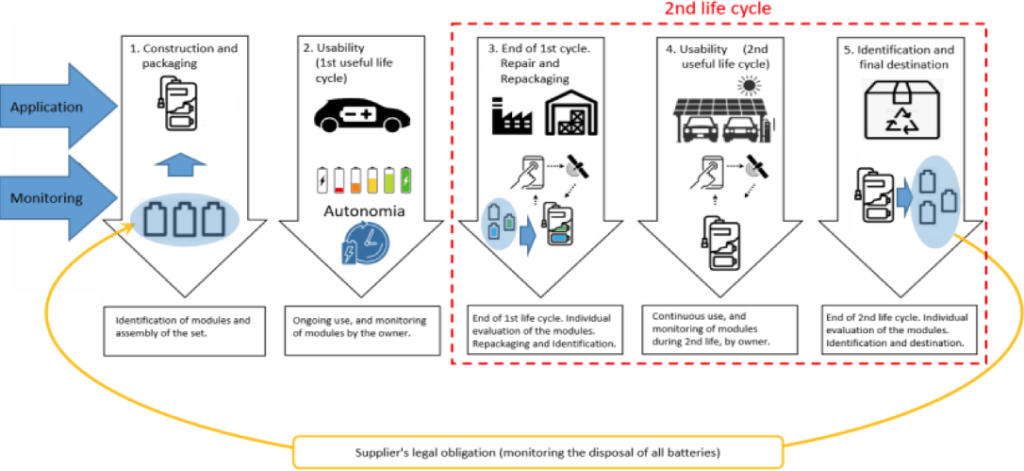
Benefits of Li-Ion Second Life Applications
The global electricity demand is estimated to have a growth rate of 2.1% per year through 2040 [6]. As a result, there is growing pressure on industry and energy providers to investigate how current energy technologies, in particular LIBs, can effectively be reused to provide further energy resources. Before the widespread adoption of second life batteries can occur both the economic and environmental benefits must be quantified.
Are second Life batteries good for the environment?
Waste management assessments have concluded that the reuse of second Life batteries is one of the most promising ventures for improving sustainability in the energy industry. LIB reuse applications have the potential to offer reductions in carbon footprint emissions, and cumulative energy demand, among other key environmental factors.
Reusing second-life batteries offers significant environmental advantages during both extraction and disposal. These benefits include reducing the need for mineral extraction and promoting resource conservation. Ultimately preventing further depletion of the Earth’s minerals and reducing the energy and emissions associated with material processing. Furthermore, the quantities of used automotive LIBs are only increasing and will present significant waste management challenges if recycling and reuse opportunities are not available soon.
Battery Recycling vs Second Life
In a Science Advances study conducted by Tao et.al, the use cases for both direct recycling and battery remanufacturing were compared to evaluate the environmental and sustainability effects of the second life application [2]. It was determined that the use case involving the second life application for the LIB significantly outperformed the direct recycling of the LIB after EV use in terms of overall environmental and sustainability effects.
Cumulative energy density and life cycle carbon footprint of Second life batteries have the potential to be reduced by 2-6% and 8-17%, respectively. When the LIB is reused in a second life application as compared to direct recycling after EV use [2]. Key environmental indicators such as fossil fuel depletion, freshwater ecotoxicity, climate change, ozone depletion, etc., and their impacts were evaluated and averaged for the two use cases as shown below. The use case involving the second life application for the LIB resulted in lower average environmental impacts for each indicator when compared to the direct recycling of the LIB after EV use [2].
How much does repurposing battery cost?
Economically, many factors are being assessed currently in academia and industry to better understand the economics of SLBs as:
- The implications of critical mineral reuse and recycling
- Future battery and electricity costs
- Performance degradation of LIBs after entering second life applications.
Costs of LIBs specifically for EV applications are expected to decline overall as the technology becomes more widespread, however, if precious metal raw material costs rise with increased demand in the near term, the second life application would be a viable option for the energy storage system industry.
It can be estimated that electric vehicle batteries are priced between $19 and $131/kWh. However, the estimated cost of a second life battery, including all expenses, falls within the range of $25 to $49/kWh. Furthermore, even with the declining LIB costs, McKinsey still estimates a price savings of between 30 to 70% for purchasers of used batteries for second life applications as compared to new batteries in 2025 [7]. Despite performance degradation, studies have shown that there are still many economical applications for second life batteries.
Challenges of Second Life Batteries
Second-life batteries are increasingly being considered for repurposing after their initial use in electric vehicles. However, this practice comes with its own set of challenges, particularly regarding safety, efficiency, and economic viability.
Battery disassembly is dangerous:
Since a retired battery pack is not ideal for direct reuse, before a retired battery pack can be certified as a second life battery it needs to be remanufactured. However, the process is very difficult, problematic, and dangerous. Retired battery packs can have inconsistency of in-pack cells. This can lead to over-discharge or overcharge, resulting in thermal runaway and explosions. The majority of this remanufacturing needs to be done manually, which is dangerous due to the possible inconsistencies.
Manually over-discharging cells can lead to electrical hazards that are particularly prevalent when not all the cells in the battery module are fully discharged. This requires technicians to carry out the battery remanufacturing process under voltage [1]. As a result trained technicians and specialized technology are required during the disassembly process [9]. Furthermore, current flow caused by a bypass can lead to strong temperature development. This only increases the risk of overheating and explosions [1]. These factors highlight the need for careful handling and safety protocols when disassembling batteries.
Lack of Automation in the Battery Disassembly Process:
The lack of automation in battery disassembly is a significant hurdle for the entire industry. Once the necessary battery checks are completed, retired battery packs proceed to the ‘disassembly’ phase. The disassembly process is labor-intensive, with as many as twenty-four manual steps required. Disassembling LIB systems is a complex, time-consuming, and costly process due to the diverse range of battery designs, flexible components, and potential hazards arising from high voltages and the chemicals within the battery [1].
Each battery cell manufacturer utilizes a distinct cell type and battery construction. This results in very little to no standardization, thus making automation almost impossible [1]. This lack of automation not only increases the time and cost of remanufacturing but also poses challenges for scaling up the process to meet the growing supply of retired EV battery packs. Fig. 5 demonstrates the process of retiring batteries.
Depending on the battery, from start to finish the disassembly process can take up to sixteen hours. Many factors influence the time to disassemble from the extent of dismantling required, to the available manpower [9]. Disassembling cylindrical cells is the most challenging, whereas prismatic cells are the easiest to dismantle [10].
Economics of Battery Reassembly:
As raw material costs continue to decrease, the cost-benefit of purchasing an SLB declines. As much as 56% of the final cost of second life batteries comes from the cost of purchasing retired batteries. Making it the highest expense to the manufacturer. Labor costs and general administration represent the second and third highest expenses. Each accounts for 13% of the total cost of battery refurbishment, as noted in Fig. 6 [9].
Qualified workers are required in the refurbishment process, which significantly impacts the selling price of SLBs [9]. With the price of first-life energy storage batteries decreasing, the use case for second life batteries diminishes due to the additional design factors and risk variabilities such as administration and labor costs.
Real World Outlook
Although second life batteries are a new topic OEMs have begun experimenting with recycling. remanufacturing methods.
Nissan’s Second Life Battery Projects
In 2022 Nissan participated in a project that consisted of 78 Nissan Leaf electric batteries in Melilla, Spain. The project used the electric vehicle batteries as a source of energy, interconnecting and storing them at Enel’s subsidiary Melilla facility. This second life project can produce up to 1.7 MWh [12]. If the power plant is disconnected from the system, the storage facility can inject energy into Melilla’s electricity grid for fifteen minutes, which is enough time to reset the system and restart the power supply [10].
4R Energy Corporation
In 2010, Nissan began exploring the solutions of recycling electric batteries. Nissan partnered with Sumitomo Corporation to set up 4R Energy Corporation. 4R Energy is in charge of repurposing old Nissan Leaf batteries. These expired batteries are sent to 4R Energy to be classified into different categories of use cases. These use cases include being used in new EV batteries, industrial machinery and energy storage, and backup power units. In addition, Nissan claims to customers that older EVs still will have value to them since their battery can be reused.
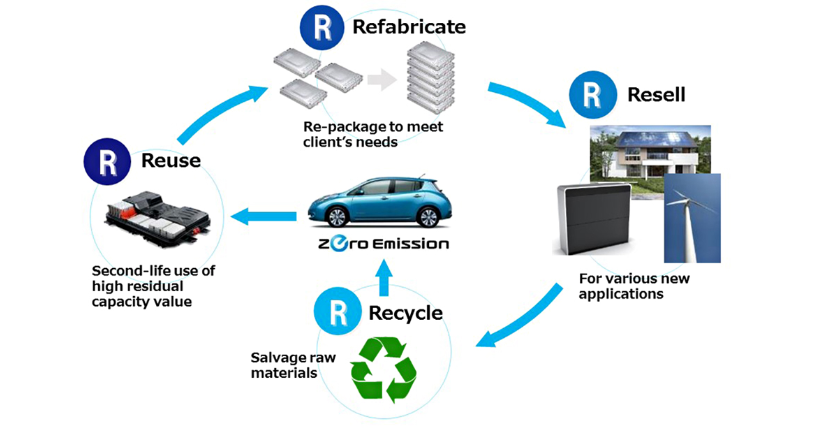
Volvo’s Second Life Battery Project
Volvo is another automotive company that is experimenting with second life applications. Volvo is currently designing a “design engine” that works with AI to determine when a battery should be replaced and how it can be reused [11]. If a battery is maintained per manufacturer specifications, Volvo claims it can be remanufactured. If this is not the case, repurposing applications include renewable energy sources such as solar and wind, power, and off-grid applications.
Current renewable energy sources are not stable, but using SLB as energy storage systems provides a promising solution due to their scalability, flexibility, fast dynamics, and most importantly, low cost. Especially in remote and off-grid areas, second life LIBs can provide power for up to a few days, while storing any extra unused energy. Volvo has also partnered with startup companies Fortum and Comsys, where SLB storage was used at hydroelectric power plants to increase the time between maintenance of turbines, and ultimately extend the battery life [12].
Bosch and BMW’s Second Life Battery Project
In addition, Bosch and BMW have been working on their second life project since 2013. Hoping to learn more about the aging process, BMW and Bosch have installed a 2800 kWh SLB system in Hamburg, Germany to support the electricity grid [13]. The cells being used are the electric BMWi3 MO/NMC-based prismatic cells. In another project, batteries have been used in Hamburg to provide power for fast-charging stations since 2014 [14]. BMW has also partnered with the energy provider Off Grid Energy to develop an off-grid energy charger that has up to 180 kWh capacity [15].
Audi’s Second Life Battery Project
Lastly, another OEM to dive into the SLB race is Audi. Audi has partnered with RWE in a pumped-storage plant in Herdecke, Germany where an energy facility uses LIBs from Audi vehicles with other systems to store around 4.5 MWh of electricity [17]. Experts have predicted that the European market for SLB will store up to 8 GWh by the year 2030 and 75 GWh by the year 2035 [18].
Future Research
The future of second-life applications for LIBs is promising and has the potential to play a significant role in sustainable energy storage and utilization [21]. Several areas of future research can further advance the utilization and optimization of second-life LIB.
Battery Performance and Degradation Analysis:
Research can be focused on understanding the degradation mechanisms and performance characteristics of SLBs under different operating conditions and applications. This includes studying factors such as cycling behavior, capacity fade, impedance changes, and calendar aging to develop predictive models and diagnostic tools for assessing battery health and remaining lifespan accurately [20].
Optimized Battery Management Strategies:
Investigation of advanced battery management strategies tailored specifically for SLB, including SOH estimation, SOC control, thermal management, and load scheduling algorithms could be a recommended route for further research. This includes developing smart charging and discharging protocols that maximize energy efficiency, prolong battery lifespan, and ensure safe operation throughout the extended life cycle of SLBs [2].
Integration with Renewable Energy Systems:
Exploration of the integration of SLIBs with renewable energy systems, such as solar and wind power, to enhance grid stability, energy storage capacity, and renewable energy penetration is another recommendation. This includes investigating optimal sizing, configuration, and control strategies for hybrid energy storage systems that combine new and SLB to address fluctuating energy supply and demand patterns [22].
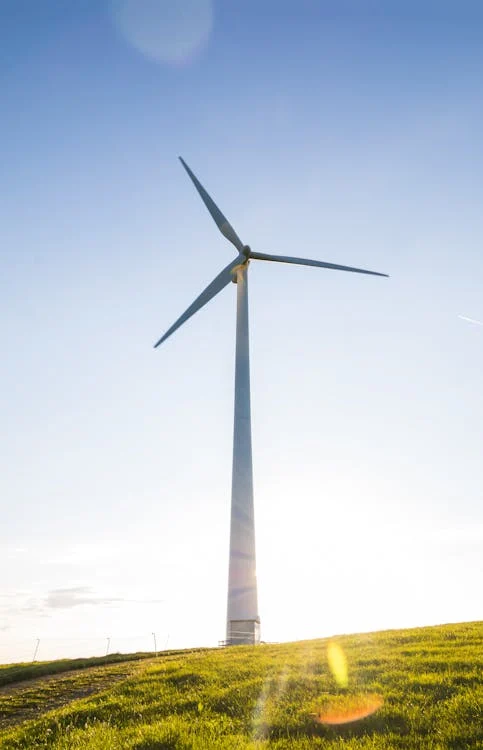
Circular Economy and Recycling Technologies:
Researching innovative recycling technologies and processes for recovering valuable materials from spent LIB, including cathode metals (e.g., lithium, cobalt, nickel), electrolytes, and conductive additives is a further suggestion. This includes developing closed-loop recycling systems that efficiently reclaim and reuse materials from SLBs, reducing the environmental impact of battery production and disposal while conserving finite resources [2].
Battery Swapping:
The use of SLBs in battery swapping stations aligns with sustainability and resource efficiency principles. As the EV industry matures, companies may explore integrating SLB into their swapping infrastructure. However, widespread adoption may take time due to technological challenges, regulatory considerations, and market dynamics. As demand for sustainable mobility solutions grows and circular economy principles gain traction, more initiatives and collaborations may be aimed at leveraging SLB in EV-related applications. Stay tuned for future implementations.
Advanced Materials and Manufacturing Techniques:
Research towards advanced materials and manufacturing techniques for designing and producing next-generation LIBs optimized for second-life applications would also be beneficial. This includes the exploration of novel electrode materials, electrolyte formulations, and cell designs that enhance performance, durability, and safety while minimizing resource consumption and environmental impact.
Conclusion
Second life LIB applications have the potential to be both a cost-effective and environmentally beneficial solution to the continually increasing global future energy demand. Many approaches are still being explored, however, several viable opportunities have emerged for second life uses, particularly for low-energy output applications such as renewable energy battery backups, grid demand stabilization, and power for charging stations.
There are still many challenges to contend with as second life applications are further investigated including the safety implications of the manual remanufacturing process, the lack of automation in the disassembly process, and future LIB cost forecasts. As recycling and reuse of LIBs continue to rise, if the energy industry can find robust solutions to the various headwinds faced with second-life LIB applications, the industry stands to realize significant cost savings and long-term positive environmental impacts.
Reference
- C. Font et al., “Second Life of Lithium-Ion Batteries of Electric Vehicles: A Short Review and Perspectives,” Energies, vol. 16.. January 2023. https://doi.org/10.3390/
- Tao, Y., Rahn, C. D., Archer, L. A., You, F., “Second life and recycling: Energy and environmental sustainability perspectives for high-performance lithium-ion batteries,” Science Advances, vol. 7, no. 45. November 2021. https://doi.org/10.1126/sciadv.abi7633
- T. Montes, M. Etxandi-Santolaya, J. Eichman, and V. J. Ferreira, “Procedure for assessing the suitability of battery second …,” MDPI, vol. 9, iss. 8. September 2022. https://doi.org/10.3390/batteries8090122
- B2U, “B2U Storage Solutions,” B2U. March 2024. https://www.b2uco.com/
- C. Rufino, E. Sanseverino, P. Gallo, and D. Koch, “Towards a business model for second-life batteries: Barriers, opportunities, uncertainties, and technologies,” Journal of Energy Chemistry, vol. 78. March 2023. https://doi.org/10.1016/j.jechem.2022.12.019
[6] International Energy Agency, “World Energy Outlook 2019,” International Energy Agency. November 2019
[7] H. Engel, P. Hertzke, G. Siccardo, “Second-life EV batteries: the newest value pool in energy storage,” McKinsey & Company. April 2019. https://www.mckinsey.com/industries/automotive-and-assembly/second-life-batteries-the-newest-value-pool-energy-storage
[8] Q. Dong, S. Liang, J. Li, H. Chul Kim, W. Shen, T. J. Wallington, “Cost, energy, and carbon footprint benefits of second-life electric vehicle battery use,” iScience, vol. 26, iss. 7. July 2021. https://doi.org/https://doi.org.2023.107195
[9] M. Shahjalal, et al, “A review on second-life of Li-ion batteries: prospects, challenges, and issues,” Energy, vol. 241. February 2022. https://doi.org/10.1016/j.energy.2021.12881
[10] Nissan, “Nissan gives EV batteries a second life,” Nissan Motor Corporation. January 2021. Available at Nissan gives EV batteries a second life | Nissan Stories | Nissan Motor Corporation Global Website (nissan-global.com).
[11] Volvo Energy, “The lifecycle of electric vehicle batteries,” Volvo. February 2023. Available at The lifecycle of electric vehicle batteries (volvoenergy.com)