Common Engineering Materials
What are common engineering materials and why are they important? Throughout history, the significant accomplishments of each era have consistently revolved around the development of new materials, playing a pivotal role in the advancement, prosperity, safety, and overall well-being of humanity. Since the dawn of civilization, it has consistently been the introduction of innovative materials that paves the way for breakthrough technologies, spanning diverse fields such as civil, chemical, construction, nuclear, aeronautical, agricultural, mechanical, biomedical, and electrical engineering.
Materials scientists and engineers remain at the forefront of various scientific domains, exerting their influence not only in the aforementioned disciplines but also in numerous other areas. The impact of materials science and engineering is palpable in our daily lives, evident each time we acquire or utilize a new device, machine, or structure.
Engineering materials encompass the assortment of materials employed in constructing artificial structures and components. The fundamental role of an engineering material is to endure applied loading without fracturing and without demonstrating excessive deflection. The main categories of engineering materials comprise metals, polymers, ceramics, and composites.
What are the 4 types of engineering materials?
The 4 main groups of engineering materials are metals metals, polymers, ceramics, and composites. Of these 4 categories, the two most common engineering materials are metals and plastics. Metals can be broken down into two main categories ferrous and non-ferrous metal alloys. Plastics can be broken down into three main categories: thermoplastics, thermosets, and elastomers.

Metals constitute the most widely utilized category of engineering materials, with metal alloys being particularly prevalent. These alloys are crafted by combining a metal with one or more other metallic and/or non-metallic substances, typically through a process involving melting, mixing, and cooling. Alloying aims to enhance the properties of the base material desirably. The compositions of metal alloys are articulated in terms of the percentages of the various elements present, measured by weight.
Ferrous alloys
Ferrous alloys, having iron as the base element, encompass steels and cast irons, standing out as the most commonly employed metal alloys due to iron’s abundance, ease of production, and material versatility. However, a significant drawback of many ferrous alloys lies in their low corrosion resistance. Carbon holds particular significance as an alloying element in ferrous alloys, where increased carbon levels generally elevate strength and hardness while diminishing ductility and weldability.
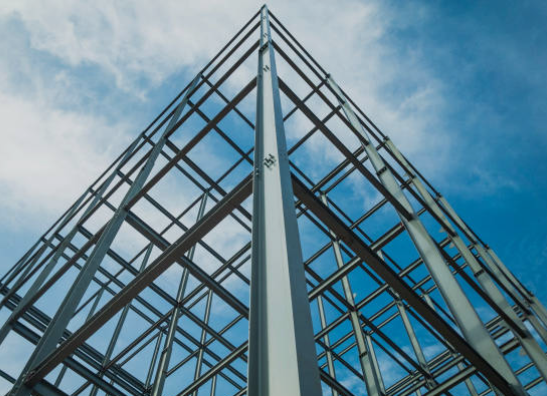
Ferrous Metals | Carbon Content | Characteristics | Common Applications |
---|---|---|---|
Cast Iron | 3-3.5% | Cheap, rusts easily, hard, good compressive strength | Anvils, vices |
Low Carbon Steel | < 0.3% | Lower strength, tough, cheap | Nails, Car bodies |
High Carbon Steel | 0.8-1.4% | Strong and tough, difficult to form | Saw blades, hammers |
Stainless Steel | ≥ 11.5% Chromium | Strong, hard, expensive, difficult to machine | Cutlery |
Non-Ferrous alloys
Non-ferrous alloys, unlike their ferrous counterparts, do not contain iron as a primary component. This diverse category includes alloys formed by combining various metals, excluding iron, and offers distinct advantages such as corrosion resistance, lightweight properties, and enhanced conductivity. Common non-ferrous alloys comprise aluminum, copper, zinc, and titanium alloys, each tailored to specific applications. For instance, aluminum alloys are prized for their low density and high strength, making them ideal for the aerospace and automotive industries. Copper alloys excel in electrical conductivity, finding applications in electrical components. The versatility of non-ferrous alloys makes them integral in numerous sectors, contributing to innovation and efficiency.

Non-Ferrous Metals | Characteristics | Common Applications |
---|---|---|
Aluminium and its Alloys | Light, soft, ductile, malleable, good conductor of heat and electricity, corrosion-resistant | Aircraft bodies, foil, saucepans |
Copper | Tough, malleable, good conductor of heat and electricity, easily joined | Wires, printed circuits |
Brass | 65% Copper and 35% Zinc, casts well, easily joined | Castings, boat fittings |
Bronze | 90% Copper and 10% Tin, tough and hardwearing | Bearings, coins, water and steam valves |
Lead | Very soft, low melting point, heavy common metal | Roof coverings |
Zinc | Poor strength-to-weight ratio, low melting point | Coating steel |
Is plastic and polymer the same?
All plastics are polymers (thermoplastic/ thermoset), however, not all polymers are plastics (elastomers). Plastic is a type of polymer that is made up of a chain of polymers. Polymers are compounds made up of several repeating structural units called monomers. The three main categories of polymers are:
- Elastomers (for example polysiloxane [Silicon] cooking utensils)
- Thermosetting polymers (for example: polypropylene [PP] bumpers/ door trim)
- Thermoplastic polymers (for example: polyethylene terephthalate [PET] water bottles)
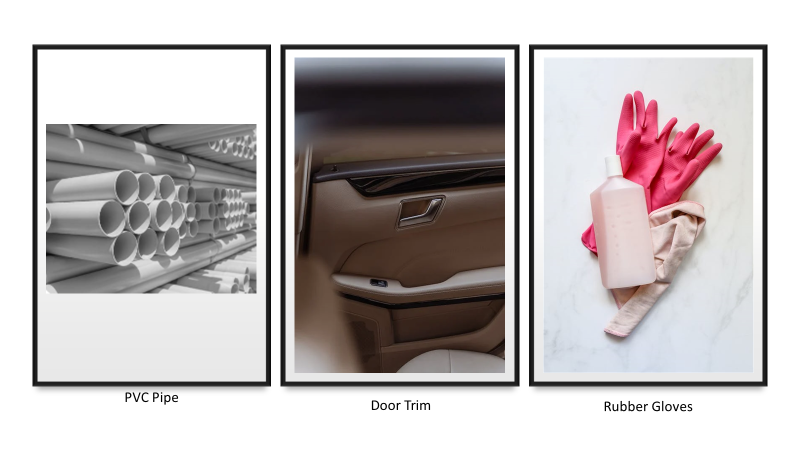
Thermoplastic polymers are categorized based on their reaction to heat, softening, and melting upon application of heat and solidifying upon cooling. These polymers, like those used in injection molding, undergo no chemical change with repeated heating and cooling unless subjected to high temperatures that break molecular bonds.
In contrast, thermosetting polymers, after initial heating, permanently harden and do not melt upon reheating. Although they can degrade under extreme heat, thermosets generally exhibit greater hardness and strength than thermoplastics, along with improved dimensional stability.
Elastomers, resembling rubber, are highly elastic polymers used in seals, adhesives, and flexible components, with vulcanization enhancing their strength and stiffness through cross-link formation.
Polymer | Type | Characteristics | Applications |
---|---|---|---|
ABS | Thermoplastic | Strong and rigid | Toys, Keyboard |
Acrylic | Thermoplastic | Transparent, hard-wearing | Plastic Windows, Bath Tubs |
Nylon | Thermoplastic | Ductile, durable | Gear Wheels |
Polycarbonate | Thermoplastic | High strength, heat-resistant | Safety Glasses, DVDs |
Polystyrene | Thermoplastic | Tough, good impact strength | Packaging, Foam Cups |
Epoxy | Thermosetting | Stiff and brittle | Circuit Boards, Electrical Insulators |
Polyester Resin | Thermosetting | Cheap, good strength and stiffness | Suitcases |
Melamine Resin | Thermosetting | Resistant to some chemicals and stains | Laminate Coverings for Kitchen Worktops |
Polyurethane | Thermosetting | Hard with high strength, flexible and tough, low thermal conductivity | Hoses, Surface Coatings, Sealants |
Vulcanised Rubber | Thermosetting | Elastic, high tensile strength, resistant to abrasion | Tires, Shoe Soles, Bouncing Balls |
Polymers Breakdown
What are the 3 methods of manufacturing polymer?
The three most common ways to manufacture polymer parts are with injection molding, compression molding, and extrusion. Thermoplastic primarily relies on extrusion (PVC pipe) or injection molding (bottle caps) while thermosets primarily use compression molding (kitchenware – bowls or cups).
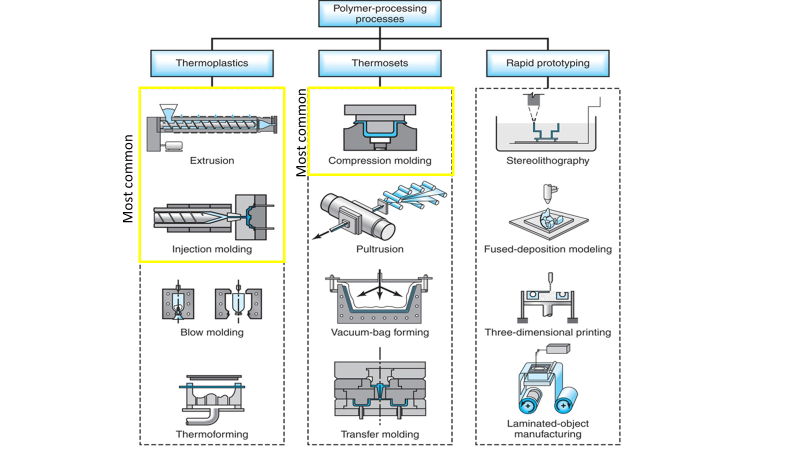
What are ceramics?
A ceramic is an inorganic solid compound made from metallic/semi-metallic material or nonmetallic elements such as oxygen, nitrogen, and carbon. Ceramics are shaped and then hardened by heating to high temperatures. Generally speaking, ceramics are hard, corrosion-resistant but brittle. The two main classifications of ceramics are :
- Crystalline ceramics:
- Traditional ceramics, such as clay
- Modern ceramics, such as alumina (Al2O3)
- Glasses – mostly based on silica (SiO2)
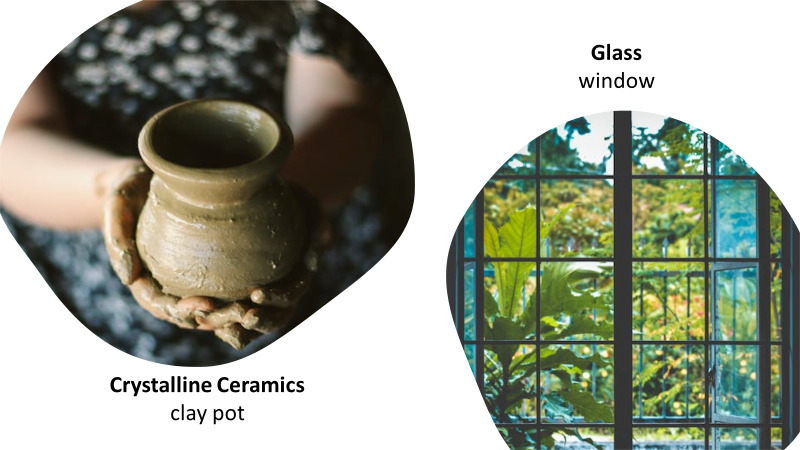
Glass:
Glass is a ubiquitous material employed in various applications such as windows, lenses, and containers. Unlike other ceramics, glasses are amorphous, primarily crystalline. Key advantages of glasses encompass transparency and ease of fabrication. Silica serves as the fundamental component, and additional elements can be incorporated to tailor its properties. Glass formation involves processes like melting and casting into molds, softening and rolling, and softening and blowing into desired shapes.
Cements:
Cements, upon mixing with water, create a paste that solidifies, allowing for shaping before hardening into firm structures. Portland cement, a common type, results from blending clay and limestone, then firing at high temperatures. Used in concrete by mixing with sand, gravel, and water, it finds extensive applications in construction. Mortar is another application when mixed with sand and water. Despite weaknesses in tension, cement is robust in compression, cost-effective, and widely utilized in constructing buildings, bridges, and large structures.
Clay Products:
Clay, a prevalent ceramic material, can be shaped and solidified through high-temperature firing after mixing with water. Structural clay products, including bricks, tiles, and piping, serve diverse applications. Whitewares, used for pottery and plumbing fixtures, constitute the other primary classification of clay products.
Refractories:
Refractory ceramics withstand high temperatures and harsh conditions, offering thermal insulation. Commonly, bricks are utilized as refractory ceramics due to their resilience in extreme environments.
Abrasives:
Abrasives are hard materials employed for cutting, grinding, and wearing away softer substances. Possessing high hardness, wear resistance, and temperature resistance, abrasives can be bonded to surfaces (e.g., grinding wheels and sandpaper) or used as loose grains (e.g., sandblasting). Cemented carbide, silicon carbide, tungsten carbide, aluminum oxide, and silica sand are typical abrasives, with diamond serving as an excellent yet costly abrasive material.
What are examples of composite?
A composite is a material that is made by combining two or more materials that are independently processed and then combined to generate superior properties than the individual materials (i.e. constituents of the composite material). Composite materials are made up of matrix material and a re-enforcing material in the shape of whiskers, fibers, flakes, or particles. The properties of a composite depend on components, the physical shapes of components, and the way they are combined to form the final material. Some examples of composites are:
- Absorbent concrete
- Carbon fiber
- Fiberglass
- Kevlar
- wood
Composite | Reinforcement | Matrix | Characteristics | Applications |
---|---|---|---|---|
CRP (Carbon Fiber Reinforced Polymer) | Carbon fibers | Epoxy resin | Extremely high strength, low density, expensive | Racing Bicycles, Helmets |
GRP (Glass Fiber Reinforced Polymer) | Glass fibers | Polyester resin | High strength, good chemical resistance, lower cost than CRP | Canoes, Water Tanks |
Plywood | Layers of wood bonded at 90° to each other | Adhesive matrix | Smooth surface, good strength, may be covered in veneer | Furniture, Boat Building |
What are the 4 types of composites?
The four primary categories of composites are:
- carbon matrix composites (CAMC), an example of CAMC would be re-entry heat shields in the aerospace industry
- ceramic matrix composites (CMCs) and examples of CMC would be gas turbines
- polymer matrix composites (PMCs) an example of PMC is fiberglass.
- metal matrix composites (MMCs) an example of MMC is AlMgSi.
What are the 4 properties of materials?
The properties of a material can be broken down into the structure of the material, mechanical properties, physical/chemical properties, and property modification. See the image below for a full breakdown of the properties of a material.

What is the structure of the material?
The structure of a material refers to the arrangement of the internal components of a material. For example the atomic bonds between atoms (metallic, covalent, and ionic) or the metal crystal/grain which makes up a metal alloy.
What are mechanical properties?
The Mechanical properties are the physical properties of a material when under mechanical loading (stresses). An examples of mechanical properties are the modulus of elasticity, tensile strength, elongation, fatigue strength, and hardness. These properties can be found by conducting tests such as the tensile pull test or the Charpy impact test.
Property | Definition |
---|---|
Strength | The ability to resist an applied force |
Tensile Strength | Maximum pulling force a material can withstand before failure |
Yield Strength | Stress at which the material reaches plasticity |
Ultimate Tensile Strength | Stress at which a material breaks |
Compressive Strength | Resistance of a material under a pushing force |
Ductility | Amount a material can be stretched while being deformed |
Malleability | Ability of a material to be deformed without breaking |
Hardness | The ability of a material to be deformed without breaking |
Toughness | The ability of a material to resist wear and abrasion |
Brittleness | Potential for a material to shatter on impact |
Stiffness | The ability of a material to withstand an impact without breaking |
Elasticity | The ability of a material to resist bending |
Plasticity | Material’s ability to permanently deform after stretching |
Mechanical Properties in Design and Manufacturing
The dilemma engineers face is mechanical properties desirable to the designer, such as high strength, usually make manufacturing more difficult.

Which are physical and chemical properties?
Chemical properties are properties that a material exhibits during a chemical reaction. Physical properties are characterized as properties that can be measured without changing the identity of the material. Some examples of physical and chemical properties are melting point, thermal conductivity or expansion, corrosion, and oxidation.
Property Type | Definition |
---|---|
Physical | Strength: The ability of a material to resist an applied force. |
Density: The mass per unit volume of a material. | |
Hardness: The ability of a material to resist wear and abrasion. | |
Ductility: The extent to which a material can be stretched without breaking. | |
Malleability: The ability of a material to be deformed without breaking. | |
Toughness: The ability of a material to withstand impact without breaking. | |
Brittleness: The tendency of a material to shatter when experiencing impact. | |
Stiffness: The ability of a material to resist bending. | |
Elasticity: The ability of a material to return to its original shape when the load is removed. | |
Plasticity: The property of a material to retain its deformed shape after the force is removed. | |
Chemical | Corrosion Resistance: The ability of a material to resist deterioration due to chemical reactions with the environment. |
Chemical Stability: The capacity of a material to maintain its chemical composition and properties over time. | |
Flammability: The susceptibility of a material to catch fire and burn. | |
Reactivity: The degree to which a material undergoes chemical reactions with other substances. | |
Toxicity: The harmful or poisonous nature of a material when in contact with living organisms. | |
Combustibility: The ease with which a material ignites and burns in the presence of a flame. | |
Oxidation Resistance: The ability of a material to resist reactions with oxygen, preventing oxidation. | |
Hygroscopicity: The tendency of a material to absorb and retain moisture from the surrounding environment. | |
pH Sensitivity: The reaction of a material to different levels of acidity or alkalinity. |
What is property modification?
The mechanical properties of many materials (primarily metals and metal alloys) can be altered by undergoing special treatment. For example, metalworking processes such as cold rolling or forging enable you to increase the strength and hardness of a metal but lower its docility. On the contrary by heat treating a metal you can release some of the internal stresses inside of a metal part and thus increase its ductility but lower the material’s strength and hardness.